The wondrous world of water
-
- from Shaastra :: vol 01 edition 03 :: Sep - Oct 2021
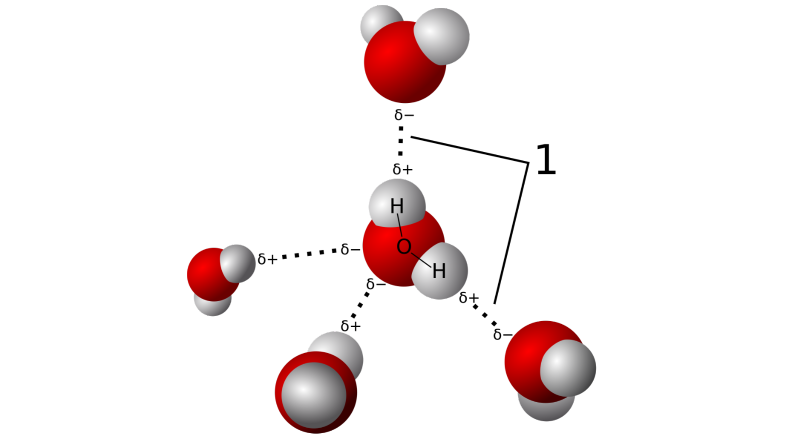
New research in India and elsewhere is turning the lens on the many avatars and possible applications of ice and water.
Alchemists have for long sought to turn lesser metals into gold. Pavel Jungwirth of the Institute of Organic Chemistry and Biochemistry, Prague, has no such plans. He'd rather create gold out of water.
Here's how. Water is an insulator in its pure state because its electrons are tightly bound to the atom. Metals, on the other hand, have electrons that move around freely. Creating a metal out of an insulator requires squeezing the electrons under intense high pressures, only available in the cores of large planets such as Jupiter.
But Jungwirth has found a way to do so on Earth. Alkali metals such as sodium and potassium shed their outermost electrons easily, making the reaction between water and an alkali metal explosive. Jungwirth put a tiny bit of water on an alkali metal drop, from which the water molecules borrowed electrons and became metallic.
"I think that's a nice trick we did," he says.
He amalgamated sodium and potassium into an alloy that flows like mercury at room temperature. He introduced water vapour in an experimental chamber while dripping drops of this silver-coloured alloy from a nozzle. The vapour mixed with the alloy droplet as the latter grew. The water vapour formed a thin layer on the droplet surface, giving it a golden sheen. "It is water, and it has metallic properties, and you don't have to go to Jupiter for that," Jungwirth says.
Water, scientists stress, is full of surprises. It can be made into metal. It can solidify into ice at room temperature or remain in a liquid form even at -40° Celsius. Normally, compounds become heavier in their solid-state. But ice floats on water.
Such anomalous properties are unique to liquid water and scientists have so far counted over 70 such traits. It is, for instance, the only compound that exists in all its three states - solid, liquid and gas - at normal terrestrial conditions. Not surprisingly, scientists are fascinated by water. They are also finding useful applications to these properties.
Take Hans Jakob Wörner, who studies the 'social life' of water molecules at ETH Zürich. He has developed sophisticated instruments and lasers that can measure a water molecule's motion within a billionth of a billionth of a second, or an attosecond. At such time-scales, only electrons can be observed moving.
To observe the motion, Wörner with his attosecond laser knocked off an electron from a molecule in distilled water and did the same with a water molecule in a gaseous phase.
The light emitted by the knocked electron in liquid water reached the detector 50-70 attoseconds later than that emitted by an electron in the gaseous phase. Photons emitted in liquid water mix and dissolve in the water, thereby struggling to reach the detector. "This shows us a direct picture of the chemical environment in liquid water," Wörner says.
He used these time delays of the solvated electron to probe into the life of water molecules and found that water exhibits a very lively social life. Water molecules are the happiest when they are connected to four other water molecules. He measured the attosecond signal for many water groups and found it increasing from a single molecule to a four-molecule cluster, which is the most stable configuration of water.
Water exhibits a very lively social life. Water molecules are the happiest when they are connected to four other water molecules.
MYSTERIOUS BOND
Then there is the hydrogen bond.
A topic very close to Arunan Elangannan's heart, hydrogen bonding is a feeble attraction between a hydrogen atom of one molecule and a highly electronegative atom like oxygen, fluorine, sulphur etc. in the other.
Oxygen in a water molecule attracts the shared electrons of the two hydrogens covalently bonded with it. This unequal sharing makes these two hydrogens attain a slight positive charge and oxygen a slight negative charge. The partial positive charge of one molecule attracts the partially negative site of another molecule, creating a bond that is called the hydrogen bond. It is ubiquitous in nature and yet scientists do not always understand it. Arunan sought to catch the deeper mysteries of these bonds. Because of its highly jumbled up picture in water, he looked to hydrogen bonding in other molecules and chemical systems for a clearer image.
He found the bond playing mischief in hydrogen sulphide gas. Hydrogen sulphide is a molecule similar to water, with sulphur replacing oxygen. The Professor at the Indian Institute of Science, Bengaluru, confirmed that hydrogen sulphide molecules exhibit hydrogen bonding.
Such a feeble bonding is rare in a gas. Unlike water, a hydrogen sulphide molecule is surrounded by 12 other hydrogen sulphide molecules.
"Physicists like to unify things. Chemists like to classify them," says Arunan, who chairs a task group formed by the International Union of Pure and Applied Chemistry to redefine hydrogen bonding. "Directionality is very important for a hydrogen bond," Arunan says.
For its many anomalies, ice has been at the centre of research, too. The most common form of ice seen on Earth - such as a glacier - floats on water, defying the norm that matter becomes solid upon increasing its mass. There would have been no life if ice sank in water, for ice forms a blanket over a water body in colder temperatures, protecting life underneath.
It has several fascinating avatars. Scientists estimate that ice can exist in over 74,000 different structural forms. Till now, scientists have discovered only 18 crystalline and two amorphous forms of ice. They name the crystalline polymorphs with Roman numerals. The ordinary ice abundant in our biosphere is Ice Ih. Not all ice structures float on water; Ice III, V and VI sink.
Ice is a complex of numerous water families and their tetrahedron homes. These homes may be spacious or packed. In many, a tetrahedral network enters into the empty space of another, making it denser than the liquid water.
In superionic ice, oxygen lets go off its hydrogens completely. Called Ice XVIII, it is black, extremely hot and can only exist at extraordinarily high pressures. It is thought to exist in the mantle of Uranus and Neptune.
Ice is found everywhere in the solar system - from the poles of inner planets and moons of the gas giants to comets. Christoph Salzmann of University College London is excited about the moons of Jupiter and Saturn. With space missions planned to these planets, he hopes to investigate the unique geysers of ice jets on Saturn's moon Enceladus as well as the icy crust of Europa and the subsurface oceans of water ice on Callisto, both moons of Jupiter.
Water at -123°C and lower exists in a 'glassy state', where it looks like liquid but behaves like a solid object. To top it, there are two forms of 'glassy states', distinguished by their densities.
Salzmann creates extreme pressure and temperature conditions in his laboratory to study how ice configures itself into a new outlook. He experimentally discovered the structures of Ices XIII, XIV, XV and is at present investigating a new form, Ice XIX.
Ice doesn't exist alone in nature but is found mixed with other elements. Ice VII, for instance, is seen in diamonds. Amorphous ice is ubiquitous in space. Salzmann has now started mixing ice with other matter. He made a comet's material by mixing buckyballs - 60-membered rings of carbon atoms - in disordered ice.
CLATHRATE HYDRATES
Scientists are also fascinated by clathrate hydrates, ice-like natural compounds in which different guest molecules are trapped in hydrogen-bonded water cages. These guest molecules can be methane, carbon dioxide, hydrogen and so on. Deep ocean floors are abundant with methane clathrates.
The energy content of methane trapped in these clathrates is higher than that in petroleum, points out Thalappil Pradeep, Professor at IIT Madras. Their extraction from the bed of the Indian Ocean can take care of India's major requirements for clean energy.
After separating methane, he finds that the leftover hydrate is a cage of cubic crystalline ice or Ice Ic. "Ice Ic is a high-density ice. It can solve many of our pure water woes," Pradeep says.
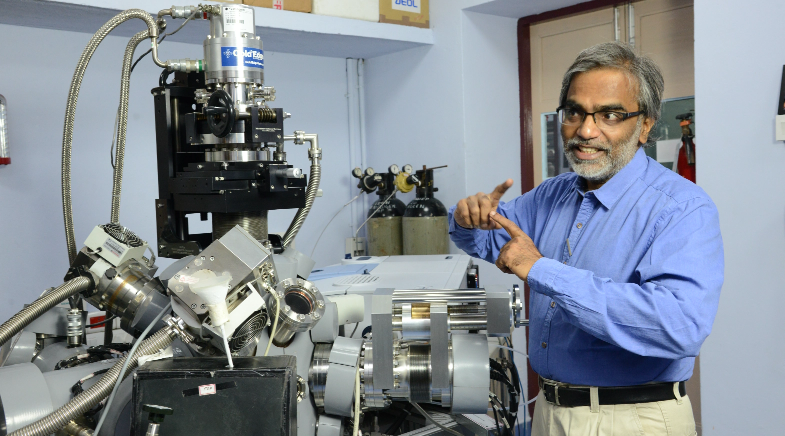
Different compounds of ice can be obtained from these clathrate hydrates, he adds. But these demand high pressure. Such conditions are readily available in continental shelves, deep ocean floors and permafrost of Earth and other moons and planets.
Pradeep's lab recently made clathrate hydrates mimicking conditions prevailing in an interstellar medium. Clathrate hydrates, he explains, can form in an ultra-cold environment where pressure is one-tenth of a trillionth of a bar. "This discovery can help us understand how chemistry happens in such adverse astronomical conditions," he says.
Water, indeed, is an unusual liquid. It is heaviest at 4°C. If cooled below that, it starts to expand. Pure water can be cooled to crystallise to -41°C. Water thriving in this metastable liquid state is known as 'supercooled water'.
Water at -123°C and lower exists in a 'glassy state', where it looks like liquid but behaves like a solid object. To top it, there are two forms of 'glassy states', distinguished by their densities. The -123°C to -41°C range is seen as an unchartered territory.
CHANGING FORMS
How water becomes ice or ice becomes water is the region of maximum anomalies, an area once considered 'No Man's Land'. "We have (now) conquered this territory," says scientist Anders Nilsson.
He prepared amorphous ice samples in his laboratory at Stockholm University and heated them by shooting an infrared laser into them. As the ice melted, it rapidly passed through many of its 'supercooled' phases, in just a matter of nanoseconds. Nilsson shot unimaginably fast X-ray pulses to probe how ice became liquid water.
What he found was baffling.
"Water exists in two different 'waters'," Nilsson says. Water under these high pressures and very low temperatures develops into two macroscopic liquids. These two 'waters' themselves are two states of matter. One of them is about 20% denser than the other. In a glass, they would seem like oil in water. "No other substance can do this," he says.
Further, at normal room temperature and pressure conditions, water shows an ice-like ordering when paraded through a carbon nanotube. Physical conditions don't allow the entry of water in carbon nanotubes. But water finds a way in by aligning its hydrogens and wagging its oxygen atoms. It enters the nanotubes in a single file, like soldiers entering a narrow burrow.
How water diffuses into a nanotube is still not clear. Sudip Chakraborty of Central University of Punjab, Bathinda, says that water molecules show mixed diffusive properties - those of bulk water as well as of the single-file chain.
Wörner's experiment will tell us how many chemical and biochemical reactions can occur in liquid water.
Chakraborty is exploring the fundamental science underlying confined water. In his computational laboratory, he traps water molecules in nanometre-sized water droplets made with organic solvents - reverse micelles - and simulates them to understand how water's behaviour changes as he increases the number of water molecules.
Water in the smallest reverse micelle, he says, is completely trapped and shows an ice-like solid structure. As he added a few molecules of water in the nano-sized droplet, it started to relax. Water molecules then attempted to move. Increasing water molecules showed a tetrahedral connectivity in the largest droplet, depicting how confined water approaches bulk behaviour.
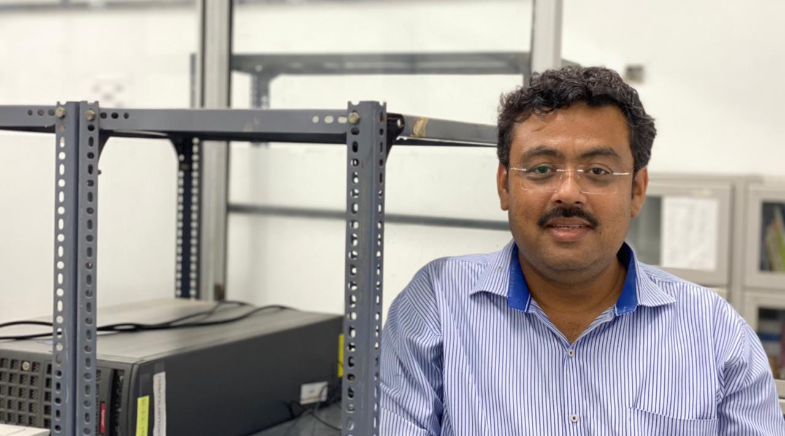
The Professor says that water molecules in these nanoconfined reverse micelles fall short of forming four hydrogen bonds. As their number increases, they get more options for bonding and try their best to stabilise themselves in a network of four hydrogen bonds. Water in its bulk tetrahedral structure shows the fastest hydrogen bond dynamics.
"A minute signature of such water is observed in the largest reverse micelle," Chakraborty says.
THE FUTURE
What is the long-term outcome of these observations? Wörner's experiment will tell us how many chemical and biochemical reactions can occur in liquid water. Water, being a very good solvent, is the template for all these reactions to happen, adds Salzmann.
The research tells us that nanoconfined drops can have potential applications in many future technologies. They can act as bioreactors for biochemical and nanotechnological reactions. Chakraborty plans to tailor these reverse micelles into drug delivery agents for future biomedical applications.
Water in many biological systems is of a confined nature. Water molecules playing around the hydrophilic sites of proteins show a unique behaviour, binding their active sites with other proteins, DNA and biomolecules.
Such research can throw light on forms of life, too. The current understanding of life is that wherever there is liquid water, there will be life. "Life can be possible on Enceladus, Europa and Callisto," Salzmann says. It can be in the oceans underneath ice beds.
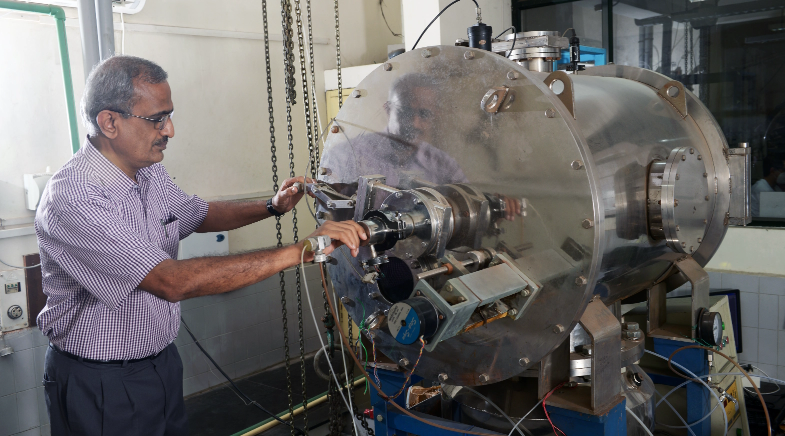
"Water is the molecule of evolution. That's why life happened in it," Arunan adds. The greenhouse effect tailored life around water. If there was no greenhouse effect and temperatures dropped below -60°C, hydrogen sulphide may then have been a good liquid for hosting life. "It is our closed-mindedness and limited experience that we can't think of life beyond water," Arunan holds.
There's no dearth of mystery - or magic - in the study of water. European researchers have joined forces to form a consortium, the Centre for Molecular Water Science (CMWS), which hopes to achieve a detailed molecular understanding of water. Nilsson says that it will attract and train younger talent in this field. For them, the glass will always be half full.
Have a
story idea?
Tell us.
Do you have a recent research paper or an idea for a science/technology-themed article that you'd like to tell us about?
GET IN TOUCH