In perfect sync
-
- from Shaastra :: vol 04 issue 04 :: May 2025
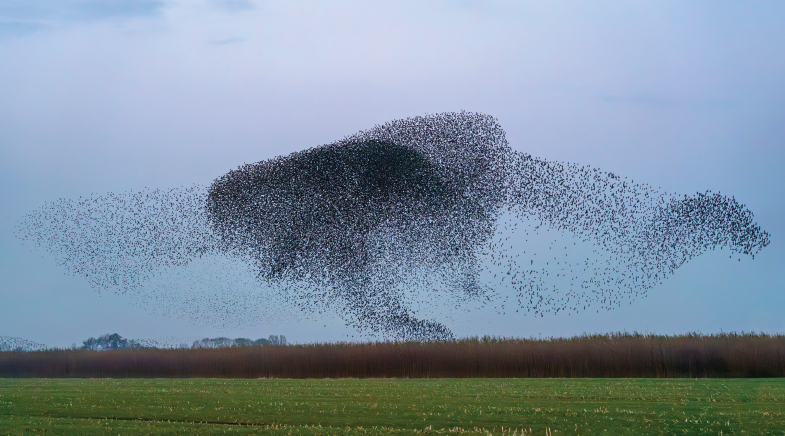
Starlings and locusts seem to make decisions in concert in a striking exhibition of groupthink. This offers clues to how complex systems work.
A monthly column that explores themes on nature, nurture and neighbourhood in the shaping of form and function.
On a busy street, a woman stops and points to something on the upper floors of a skyscraper. Soon, she is joined by more people. They all start gazing intently at where she is pointing, and continue to do so even after she stops. Then someone says, "This way," and marches off, prompting the crowd to move in that direction. Such stories are standard in comedies and comic strips, but real life can mimic art. The way crowds form, move, and dissipate has aroused researchers' curiosity. The study of collective behaviour also extends to collective thought or groupthink. How do groups of humans, from small communities to large societies, sometimes share one strong collective view on some issues, bordering on the self-destructive?
Collective behaviour is, of course, seen in species other than humans. Lions hunt in tribes, and antelopes escape in herds, each individual seeking power or safety in numbers. Termites work collectively, with no one in charge, to build nests of complex architecture with exquisite air conditioning. Fish gather together as schools to seek food or to escape, and dolphins can corral these schools, like sheepdogs do to sheep, to drive them into becoming their food.
DUCKS IN A ROW
Biologists who study collective behaviour ask three kinds of questions. What is the adaptive advantage of the behaviour? How can the ability to participate in collective behaviour be made possible during animal development? How does the collective's behaviour emerge from the individual's properties? Newborn ducklings can be imprinted to regard you as their mother if you are with them soon after their hatching and you look after them. Vulnerable ducklings are safer following their mother; that's the adaptive advantage. To do this, they must recognise their mother at birth. Here, the first visual cues seem essential. How they waddle together, with the mother duck in the lead, is an example of the emergence of a collective movement which we try to understand. Does the mother signal to each duckling or the one closest to her? Does a duckling communicate with its nearest neighbours or with others, too? Does it follow its mother even if she is at a distance with intervening ducklings? The answer to this will help us understand how collective behaviour arises from the properties of individuals.
Collective phenomena are not a prerogative of the living. We see them in the inanimate world, too. When you heat water for your morning coffee, the water comes to a boil, not in parts but as a whole. Sitting outside a little later, if you look upwards, you see clouds of water vapour staying together as they traverse the sky. The formation of clouds is widely recognised as a "collective phenomenon". Clouds emerge not from the behaviour of a single water droplet or vapour molecule, but from the interactions and coordinated dynamics of water droplets and atmospheric processes. This involves water vapour condensing onto tiny aerosol particles, forming countless microscopic droplets or ice crystals that make up a visible cloud. These droplets interact, and the presence and behaviour of each droplet influence the growth and transformation of others, ultimately resulting in large-scale cloud structures.
Physicists who examine collective phenomena through experiment and theory try to see if common principles apply to complex phenomena, and the collective behaviour of lifeless matter can be generalised to apply to living matter. Giorgio Parisi, the Nobel Prize-winning physicist, has studied the mesmerising flight patterns of starling flocks known as murmurations.
Studying starling flocks presented a unique challenge that required innovative research methods, which Parisi and his team developed in the early 1990s, installing multiple high-precision commercial cameras on Rome's rooftops. These cameras were synchronised with millisecond precision to capture each bird's three-dimensional position and velocity in flight. This technical achievement allowed the research team to transform qualitative observations into quantitative data suitable for rigorous mathematical analysis. Till then, photographs gave only one view of the murmuring flock. Parisi's research revealed that starling flocks operate according to principles that appear counterintuitive at first glance. Unlike many systems where order breaks down as size increases, starling flocks maintain exceptional coordination regardless of their population. The coordination doesn't rely on hierarchical leadership or centralised control mechanisms that might be expected in such well-orchestrated movements. There is no mother duck.
HOW THEY FLOCK TOGETHER
The most remarkable discovery in Parisi's research was identifying how flock-size independent collective movement occurs within starling flocks. This phenomenon represents the mathematical foundation of how thousands of birds move as if they were a single entity. When one bird changes direction or speed, this information propagates instantly throughout the flock. The result is a system where behavioural influence doesn't diminish with distance as rapidly as expected in traditional physical systems. Contrary to what might be intuitively expected, Parisi discovered that starling interactions depend not primarily on the metric of distance but on 'topological connections'. Each bird interacts with and responds to its nearest neighbours rather than all birds within a certain physical radius. Also, the interactions are not dependent on the density of the flock. This interaction pattern creates a network where information travels efficiently throughout the system instantly. In Parisi's words, "The interaction between starlings depends not so much on the general distance between them as on the connections between the closest birds." This finding helps explain how a flock can maintain coordination across vast spatial dimensions without requiring each bird to track the position of every other member.
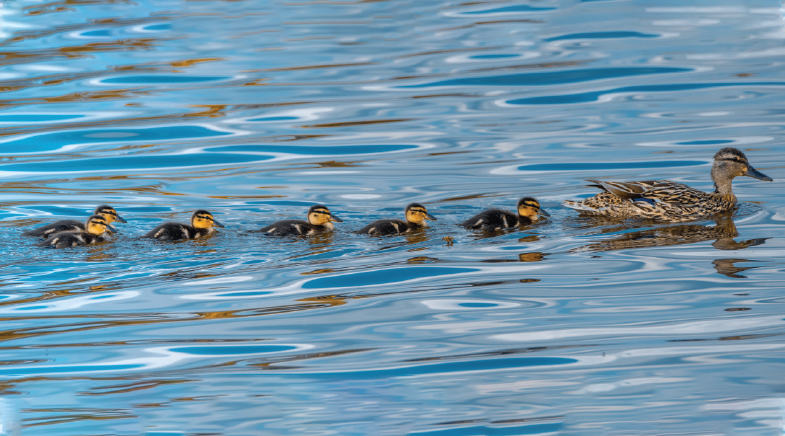
The mathematical models developed by Parisi reveal that these flocks exhibit properties similar to physical systems at phase transitions, where correlations become long-ranged and the system becomes extraordinarily sensitive to small perturbations. The flock's behaviour transcends the capabilities of any individual bird, creating what scientists call "emergent phenomena" – collective behaviours that cannot be predicted by studying individual components in isolation.
What is particularly fascinating is that starling flocks maintain this critical state without external tuning. Unlike many physical systems that require careful parameter adjustment to reach criticality, these biological collectives naturally position themselves in configurations that optimise information transfer and collective response. Parisi explains that the mesmerising complexity of starling murmurations emerges from straightforward behavioural rules followed by each bird. The collective motion arises not from sophisticated individual intelligence but from the networked interaction of many individuals following basic protocols. This exemplifies a core principle of complexity science: an increase in components creates changes that are not merely quantitative but qualitative, fundamentally transforming the system's behaviour.
Does the mother signal to each duckling or the one closest to her? Does a duckling communicate with its nearest neighbours or with others, too?
In his book In a Flight of Starlings: The Wonder of Complex Systems (reviewed in Shaastra magazine: An ode to complexity), Parisi uses murmurations as an entry point to explore broader principles of complexity that appear across diverse phenomena in physics, biology, and even social systems. Despite apparent differences, he demonstrates that many complex systems share underlying mathematical principles, revealing surprising unity in seemingly unrelated natural phenomena.
SYNCHRONISED SWARMS
When we think of animal teamwork, images of starlings and other birds flying in formations or fish swirling in tight schools come to mind. But perhaps the most awe-inspiring, and sometimes terrifying, example from nature is the swirling, rolling, and seemingly unstoppable march of a locust swarm. These vast armies of insects, stretching for miles and numbering billions, can devastate landscapes in hours. But how do so many tiny creatures synchronise their movement perfectly, without a leader or apparent communication?
In a groundbreaking study published in Science in February 2025, a team of researchers led by Iain Couzin's group in Germany set out to answer this longstanding biological mystery. They cracked open the black box of collective locust behaviour through a clever mix of fieldwork, high-tech and mathematical modelling. Some aspects are similar to those of starlings, others are very different.
The desert locust, Schistocerca gregaria, is infamous for its swarming phase. Usually solitary, these insects can form gigantic groups called swarms under certain environmental conditions (such as after heavy rains). In full force, these swarms move together with mesmerising uniformity, and every individual turns, accelerates, or stops in a coordinated manner. For decades, scientists have speculated about possible explanations. Maybe locusts communicate through smells or sounds. Maybe there's a leader or "command centre" insect. Or perhaps they follow each other in a chain reaction.
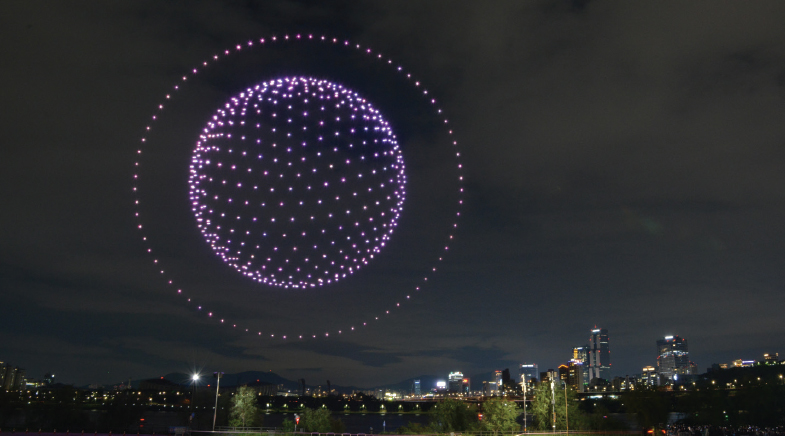
To understand swarming as it happens in real life, the research team travelled to areas where locust swarms naturally occur. Here, they observed and recorded the movements of individual locusts in the chaos. They used high-resolution video, Global Positioning System (GPS) tracking, and even miniature insect sensors to map their paths. What they saw was remarkable: while the swarm moved in one direction, the locusts weren't blindly following the crowd. Instead, each individual constantly adjusted its course in response to just a handful of nearby neighbours it saw. If a locust on the left sped up, the others sped up. If one turned right, the closest followers did, too. This ripple of coordination spread across the swarm, keeping the mass moving as one. There was no evidence of leaders, central control, or sophisticated communication. Instead, local interactions ruled the day.
Fieldwork gave the researchers raw data, but they needed to create controlled environments to test their ideas truly. Here, they used virtual reality (VR). In the laboratory, locusts were placed on tiny, treadmill-like platforms called "trackballs". Surrounding video screens displayed moving images of other locusts, making it seem like the real insect was in the middle of a swarm. By changing what the locust "saw" on the screens, the researchers could precisely measure how a locust reacts to the movement of virtual neighbours. The results were consistent. Each locust aligned its movement almost instantly with that of the nearest virtual "locusts". This alignment was most substantial when the neighbours were within a certain distance (not too close or far). The locusts didn't follow blindly; if a neighbour went the wrong way, the locust would ignore it, using information from other nearby locusts. Each insect used a mental filter, focusing only on a few reasonable, nearby visual cues (not touch or smell!). The rules were to align with your immediate neighbours. Ignore neighbours who are too distant or moving erratically, and adjust your speed to match those around you. The team also built huge arenas, tens of metres in diameter, where they observed swarms with individuals identifiable with machine learning algorithms.
The simplicity and efficiency of swarming behaviour have inspired engineers to design fleets of drones, robots, and self-driving cars. By mimicking starlings' or locusts' "local rules", these machines can coordinate with each other without central control, making them more reliable and adaptable in unpredictable environments. Swarming behaviour theory has also been applied to the collective movement of cancer cells during metastasis. And the same principles seem to apply. Sometimes there's a mother 'duck' that the ducklings (other cells) follow. At other times, the cells behave like locusts, 'sensing' their neighbours, but acting as a collective. Complex phenomena theory is also being used to analyse how decisions are made in concert by thousands of different kinds of neurons in our brain. In biology, each case needs study to decipher what happens, but from the collective behaviour of cancer cells, to our brain, to how we end up stampeding, many general principles of phase transition, akin to the boiling of water, seem to apply.
Also Read/Watch
Parisi, G. In a Flight of Starlings: The Wonders of Complex Systems. amzn.in/d/aQjwzN8
Sercan Sayin et al. The behavioral mechanisms governing collective motion in swarming locusts. Science, Vol. 387, No. 6737 (2025). bit.ly/locust-collective
Collective Intelligence in Animals and Robots. bit.ly/couzin-collective
Have a
story idea?
Tell us.
Do you have a recent research paper or an idea for a science/technology-themed article that you'd like to tell us about?
GET IN TOUCH