Susanne Mandrup on fat cells and human health
-
- from Shaastra :: vol 03 issue 04 :: May 2024
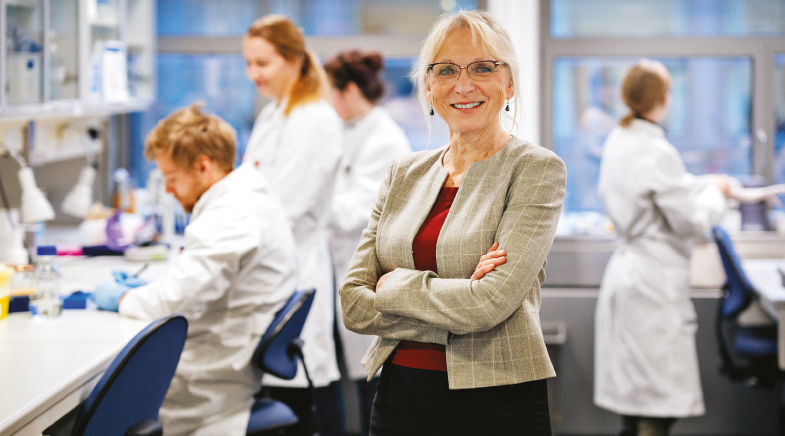
Biochemist and molecular biologist Susanne Mandrup on the importance of studying fat cells.
In the landscape of biological mysteries, fat cells loom large. And Susanne Mandrup is looking to solve that mystery with the diligence of a forensic 'fat investigator'. Mandrup, Director of the Center for Functional Genomics and Tissue Plasticity, and of the Center for Adipocyte Signaling, at the University of Southern Denmark, is peeling off the layers of complexity of fat cells to understand their profound impact on human health. She was the first to show how the structure of genetic material inside cells, called chromatin, changes as fat cells develop (bit.ly/Chromatin). This established how fat-cell-specific features are established. For her lifelong work on understanding fat cells, Mandrup was in January 2024 given the Villum Kann Rasmussen Annual Award in Science and Technology, one of the largest Danish research awards. Excerpts from an interview to Shaastra:
What triggered your career interest in fat cells?
I've always been interested in understanding how genes are regulated. When I started looking at DNA in high school, I thought I need to understand how that is translated in the cell to become the basis for life. Studying fat cell differentiation, we can learn general aspects of stem cell differentiation and how gene regulation is coordinated to switch on specialised gene programmes in cells. In particular, we can understand how fat cells are developed. It turns out that fat cell development is extremely important for human health. We need fat cells, and (need) them to function properly, in order to be healthy.
What do we know about fat cells in humans?
We know they're important. Genetic variations that cause dysfunctional fat cells, so-called lipodystrophy, lead to metabolic diseases. Similarly, a lot of evidence points to fat cell dysfunction in obesity as a major driver of the metabolic complications associated with obesity. There's genetic predisposition to develop obesity complications; but it appears that you can be obese in a very unhealthy way or in a less unhealthy way. Less unhealthy obesity is associated with fat cell function genes.
What are the gaps your work looks to fill?
Our work is curiosity-driven and targeted at understanding fat cell development, fat cell function, and how fat cells function – not in the Petri dish, but in the natural environment, in the adipose tissue. There's no direct medical application of that, but it will lay the groundwork for future translational work. We have one study out where we looked at the mouse adipose tissue at single-cell resolution, and how it changes in obesity. Now we are working on both mouse and human studies looking at what happens when during weight loss: is there a lasting memory in the adipose tissue that we should be aware of, which maintains some dysfunction? Or can we direct the adipose tissue to become more functional, more metabolically active? What are the genetic variants in people that could lead to more rapid loss of dysfunction memory versus a slower loss of that memory?
"Fat cell development is important for human health. To be healthy, we need fat cells, and (need) them to function properly."
In your Nature Genetics study (bit.ly/EnhancerCommunity), you looked at enhancer communities. What did it establish?
These regulatory sequences in DNA, called enhancers, function via the ability to recruit many different regulatory proteins called transcription factors and co-factors. By means of these big protein assemblies, enhancers can increase or decrease the expression of genes. We showed that enhancers form communities with other enhancers that come together in 3D, communicating with each other and enhancing each other's function. We showed that this cooperativity is important for the regulation of nearby genes.
In this context, what does 'expression of genes' mean?
In the DNA strand, you have a gene encoding a heritable trait. In order to express that trait, the gene needs to be copied to RNA. This RNA is then spliced by cutting out the parts that are not needed for the transcript. The spliced RNA is exported from the nucleus to ribosomes in the cytoplasm where it is translated to a protein.
How is gene expression related to comorbidities in obesity, and how is it related to diseases?
For a fat cell to function properly, it needs to express enough of those genes that make it able to handle fat, respond to signals and send out signals itself. Take insulin, an important hormone in adipocyte function: it tells the fat cell to take up more sugar and fat. And if the fat cell cannot respond to insulin, it will not be stimulated to take up fat and sugar, which will then go to other cells – like liver cells and muscle cells. This leads to dysfunction in these tissues. So, we need fat cells to extract the sugar and the fatty acids from the bloodstream. We also need fat cells for releasing hormones signalling to other tissues. It's important to know how fat cell genes are regulated because they're important for having healthy fat cells that can protect you from obesity comorbidities.
Are fat cells the same in different species (mice or humans) or genders or races?
They're similar, but they also have differences. Even fat cells in different depots in the same individual are slightly different. Overall, they have similar gene programmes and respond similarly to hormones, but the magnitude of the effect can differ between people. That may have to do with developmental differences, something that's laid down already in the epigenetics when fat cells develop, but it could also depend on the exact environment that the fat cells see. That's why it's important to study fat cell function not just in the Petri dish, but also in the living organism in the different depots. We're comparing all the cells in the adipose tissue with single-cell technologies, and we're comparing different depots to see how they respond, for example, to obesity or to dietary challenges.
What about diet?
One of our recent (but as-yet-unpublished) studies shows that if you expose mice to an unhealthy, very high-fat diet, there is an almost immediate response in how the fat cells read their gene programmes and express their genes. The fat cells dynamically respond to diet and, we think, try to adapt to the diet. That plasticity of fat cells is probably important for our metabolic health.
Have you looked at exercise?
I have not, but other colleagues in the field have. With extensive exercise, our fat cells begin to release fat, which then goes to the muscles. So, there are metabolite effects, but there are probably also signalling molecules from the muscle cells that regulate fat cells.
What technologies are the most valuable in advancing the understanding of fat cells?
The introduction of high throughput sequencing or Next Generation Sequencing has been a game-changer. We acquired one of the early Illumina sequencers and started doing genome-wide sequencing in adipocytes, not to sequence the entire genome but to sequence the functional one. For example, these regulatory sequences in fat cells, to see how they were switched on and off.
Another big game-changer was the introduction of single-cell sequencing. We can take out a biopsy from an animal or from a patient, and then understand how genes are regulated in the individual cells. We can also begin to understand how the epigenome is differently regulated in individuals. It's been a game-changer in the fields of cell biology and molecular biology. Instead of mostly studying molecular functions of cells in Petri dishes now, we can begin to study cellular functions in vivo, in living animals or in patients. We are taking out a biopsy and measuring, at a certain point in time, how the different cells are expressing their genes. In doing so, we get an account of all the individual cells in the biopsy.
The next frontier is to study molecular mechanisms in real time in living cells so that you have an account of the plasticity over time: at one point, a cell expresses a certain set of genes and, at a later point, it expresses slightly different gene programmes or changes its signalling capacity.
How might our understanding of fat cells change over the next decade?
I'm pretty sure that 10 years from now, we'll understand much better how genome variation in people affects fat cell differentiation and function and, therefore, the predisposition to develop metabolic diseases. That will give us some guidance as to how we should live, or give advice, for instance, that your fat cells have a predisposition to store more fat in the abdominal region rather than in the subcutaneous regions. That means you should be more careful.
What does your study on different body types – apple-shaped or pear-shaped – establish?
It compares abdominal fat with subcutaneous fat. A pear shape is associated with much better metabolic health; it's been proposed that it's because the fat cells in the lower body depot hold on to the fat more. So, there's less fat in the circulation. The ability to expand those depots to sequester the fat in a safe place seems to be associated with better metabolic health. We'd like to understand how that functions at a molecular and cellular level. We're studying both depots at single-cell resolution; we're also studying how the depots are affected by obesity. We're comparing individuals with lower/higher BMI to see how the functioning of these fat tissues is affected by shape and by BMI. We hope to get a better understanding of why some people have more well-functioning fat depots; what gene programmes are different; and what signalling pathways are different between people.
Have a
story idea?
Tell us.
Do you have a recent research paper or an idea for a science/technology-themed article that you'd like to tell us about?
GET IN TOUCH