The Indian effort to decode the SARS-COV-2 virus
-
- from Shaastra :: vol 01 edition 01 :: May - Jun 2021
Indian biologists are working to understand the coronavirus better, and are hoping to use that knowledge to develop better vaccines for the future.
THE CRYO ELECTRON Microscope (Cryo-EM) is rarely seen by the public as a weapon to fight the COVID-19 pandemic. Costing nearly ₹50 crore and requiring an additional ₹30 crore for infrastructure, it is among the most coveted pieces of equipment in modern biology laboratories. Its high price meant that Indian laboratories have been slow to get the machine. The National Centre for Biological Sciences (NCBS) in Bengaluru got the first state-of-the-art Cryo-EM in the country, but several more are on the way now: at the Indian Institute of Science (IISc) in Bengaluru, the Centre for Cellular and Molecular Biology (CCMB) in Hyderabad, and at IITs in Delhi, Chennai and Mumbai.
At IIT Delhi, virologist Manidipa Banerjee will get her new machine later in the year: she was supposed to have got it last year, but it’s been delayed by the pandemic. IIT Delhi has an older machine but the new one is more powerful and lets scientists see proteins from up close, with a clear view of where smaller molecules attach to them. For Banerjee, the Cryo-EM is an invaluable tool to see how antibodies dock to viruses. By watching how antibodies and viruses lock on to each other, scientists can determine vulnerable spots in the virus.
A state-of-the-art Cryo-EM operates at 300 kilovolts (kV). Banerjee’s older machine works at 200 kV, but it was good enough for her to do some work on the SARS-CoV-2 virus. Using it along with a more modern one at the national Cryo-EM facility at the NCBS, she has developed a vaccine candidate and a platform technology. “The chance of another pandemic is high,” says Banerjee. “We should have multiple kinds of technologies so that we are ready the next time something happens.”
In major laboratories around the world, scientists are using advanced equipment and deep science to understand the structure and behaviour of the SARS-CoV-2 virus.
COVID AS CATALYST
India, with a comparatively medium-sized scientific ecosystem, has not been in this game except in small ways. The COVID pandemic, however, has induced some scientists to adapt their work to future vaccines. While it may take some time for their research to result in commercial products, they are laying the groundwork for next-generation vaccines in the country.
There is never a good time for a pandemic, but the year 2020 was perhaps a better time than any other to deal with one. A number of technological advances that happened over the past decade helped scientists to rapidly sequence the virus, look at it from close quarters, and get vaccines ready very quickly. However, the fight against COVID and other viruses will go on for a long time. So scientists are using even deeper science and more advanced technology to develop vaccines that will make their way into clinics over the next few years.
Cryo-EM is one of the most recent and significant of these developments. Next-generation sequencing is another piece of technology that has completely changed the approaches to viruses and vaccine discovery. Although developed more than a decade ago – commercial systems have been available since 2005 – next-generation or massive parallel sequencing has been gaining in power over the last decade and has been used in ingenious ways to figure out the stability of proteins, an important piece of information while designing antibodies against viruses. The development of techniques to make mRNA vaccines was another recent advance, and so are methods to make adjuvants that help vaccines perform their tasks more efficiently. There are many other precise techniques that are now being tailored to study viruses and how they attack the host.
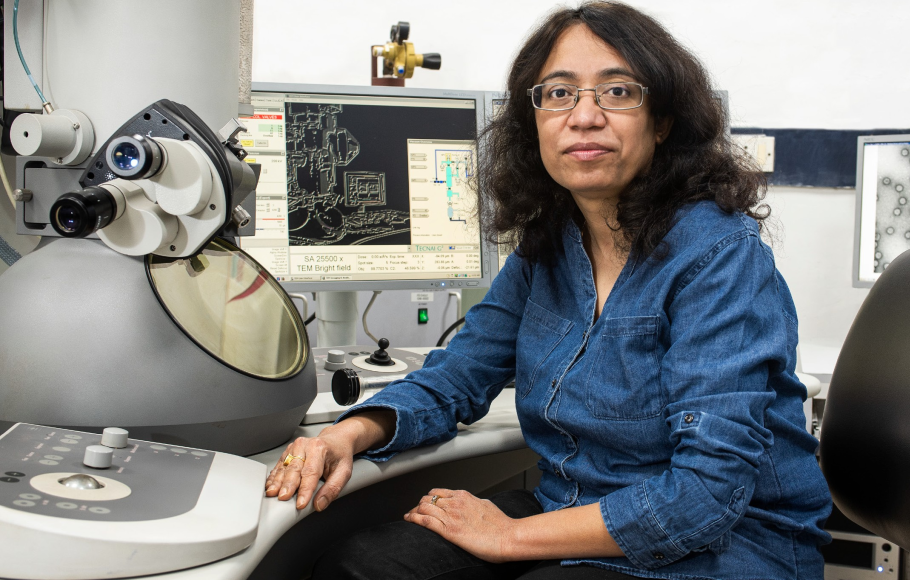
Banerjee’s primary interest is on how viruses assemble themselves and gain access to the host cellular machinery, a useful piece of knowledge in designing foolproof vaccines. At IISc, structural biologist Raghavan Varadarajan at the Molecular Biophysics Unit (MBU) works on protein structure and stability; this knowledge, too, is invaluable in designing stable proteins that can work as vaccines. At IIT Kanpur, Dibyendu Kumar Das studies how the virus changes conformation – the way its proteins are arranged – before entering the host cell, and how this conformational change is important for breaking into the host.
At the Centre for BioSystems Science and Engineering at IISc, Siddharth Jhunjhunwala works on engineering immune responses to viruses and other invaders. By studying how materials interact with the immune system, he could develop adjuvants that improve the efficacy of vaccines. After the COVID-19 pandemic, all these scientists redirected their research towards developing vaccines at some time in the future.
THE CRYO-EM EDGE
The Cryo-EM won its developers a Nobel Prize in 2017. One of them, Richard Henderson of the Molecular Research Laboratory at Cambridge, was closely involved in setting up India’s national Cryo-EM facility at NCBS in 2017; his student Vinoth Kumar moved to NCBS as part of the ‘package’. The Cryo-EM solved a major problem that structural biologists faced: to figure out the structure of a protein using x-rays, a method known as x-ray crystallography, they had to get the protein to crystallise. This is often hard – and, in some cases, impossible. Cryo-EM got around this problem by rapidly freezing the sample, letting biologists see proteins at high resolution.
There are 17 varieties of the influenza virus and only four are circulating in the human population. But the others have the potential to spread – with much higher fatality rates than SARS-CoV-2.
Technologies for the Cryo-EM had been under development for two decades, but the technique became mainstream only early in the last decade. Virologists were among the first to use this technique as it let them see the antibody and the virus attached together. The microscope gave them a tool to look at different parts of the virus and see which protein can trigger an immune response, and how antibodies attach themselves to the protein on the virus surface. Such techniques were used by vaccine makers in the early days of the pandemic to develop vaccines. Now they are being used to develop vaccines that provide more generalised immunity, including against new mutated forms of viruses.
There are several methods to provide generalised immunity. One is to have multiple proteins from the viral surface, so that at least one of them will work against mutated forms. A second approach is to look at parts of the virus that do not change during mutations, and then use that part to develop a vaccine. It is a hard problem to solve, as the unchanging parts are usually buried deep inside – and so not easily accessible to the immune system. Both these methods are being tried now for COVID-19.
Banerjee uses the first approach of using multiple particles on the viral surface. She was on the verge of starting animal trials with one such vaccine, consisting of four protein particles, in collaboration with the Translational Health Science and Technology Institute in Delhi, when the second wave of the pandemic hit the country.
At IISc, Varadarajan is trying to use the second approach, looking for proteins that do not mutate easily, to develop vaccines for both COVID-19 and influenza. Such a combination vaccine may be necessary sometime in the future. Epidemiologists consider respiratory viruses as among the biggest threat to public health, and coronaviruses and influenza viruses are among the most threatening respiratory viruses. The influenza virus exists in 17 varieties, but only four are circulating in the human population at the moment. Reservoirs exist for the remaining varieties, and they have the potential to spread in humans without any warning with much higher fatality rates than SARS-CoV-2.
The holy grail of vaccine research, though, is still far away: a vaccine developed within weeks or days of a pandemic, which can work against any mutated form of the virus.
Varadarajan does not use a Cryo-EM to find the protein structure. Instead, he looks for mutations in the amino acid sequence of the virus, especially for the so-called correlated mutations, which are changes in two amino acids at the same place in two different proteins. By building a library of correlated mutations and comparing them, scientists are able to figure out the configurations of proteins and predict the structure. Alphabet subsidiary DeepMind uses correlated mutations to predict protein structures. In 2012, Varadarajan was among the first few biologists in the world to use rapid sequencing to judge mutation rates and analyse these mutations to figure out the structure of proteins. Now he uses this technique for vaccine development.
When biologists have a single protein, Cryo-EM remains the most powerful technique that biologists can use. Varadarajan does not need this technique so much because he looks at the structures of a large collection of proteins. His aim is to figure out mutations in the virus that result in stable proteins. In other words, his interest is not in a specific structure but in finding methods to stabilise a specific sequence of amino acids. Proteins on the surface of viruses are stable only for short periods, and so it is not easy to use them for generating antibodies. Which is why all the attention of vaccine developers is focussed on the stable spike protein.
STABILISING THE PROTEIN
Molecules other than the spike protein could be developed as vaccines if scientists could find a way of stabilising them. Stabilising the protein is also important in preventing the virus protein in changing conformation, a process that is important for it to move inside the cell. Varadarajan is using his knowledge of protein stability to develop a combination vaccine for COVID-19 and influenza (See ‘This vaccine can take the heat’). “If the virus has a large and stable protein, vaccines are more likely to get a better antibody response,” says Varadarajan.
By using new, stabilised proteins on the viral surface, he hopes to develop vaccines that can work in mutated forms of the virus. One day, using such principles, biologists like him hope to develop a single vaccine that can work against all forms of influenza. It is one of the hardest problems for virologists to crack.
For a coronavirus, the lack of stability of surface proteins may be part of a strategy to penetrate the host membrane. For example, many viruses, including SARS-CoV-2, go through a change in the conformation of proteins in their surface just before entering the host cell. Cryo-EM has provided good images of conformations before and after the entry into the cell, but they are static images. Cryo-EM uses frozen samples, and so cannot watch the spike protein go through the conformational change. This is done through another technique called Fluorescence Resonance Energy Transfer (FRET), which Das uses at IIT Kanpur in combination with powerful microscopes.
FRET uses energy transfer between two fluorophores – compounds that can emit light when excited – to measure the distance between them. Till early this century, it had been used only to measure average distances in collections of molecules. Single-molecule FRET used similar principles to measure distances between parts of a large molecule like proteins. In 2014, James Munro at the Tufts University School of Medicine in the US used it to understand the conformational changes of HIV protein as it entered the host cell. In 2017, Das moved to Tufts University to work with Munro on the HIV virus as a post-doctoral scholar. Das and Munro showed in 2018 that the HIV envelope was intrinsically dynamic.
Das moved to IIT Kanpur in 2019 to start his own laboratory, where he studied the Ebola virus and SARS-CoV-1 virus. When the COVID-19 pandemic started, he switched to the SARS-CoV-2 virus. Its structure is not too different from that of the older SARS virus. Using FRET, he now tracks the conformational changes of the spike protein in the SARS-CoV-2 virus as it enters the cell. Once the intermediate forms of the spike protein are mapped out, scientists can look for more vulnerable spots on the protein, apart from what are obvious before the change in conformation.
It is a big jump in understanding the virus. “It is one thing to see that a huge structural change has happened,” says Das, “and quite another to map out the trajectory.” Once the intermediate stages of the change are clear, scientists will have more sites on the spike protein to exploit. It may be that some of them are more resistant to mutations and so more vulnerable to vaccines.
Have a
story idea?
Tell us.
Do you have a recent research paper or an idea for a science/technology-themed article that you'd like to tell us about?
GET IN TOUCH