A wobble has physicists astir
-
- from Shaastra :: vol 01 edition 01 :: May - Jun 2021
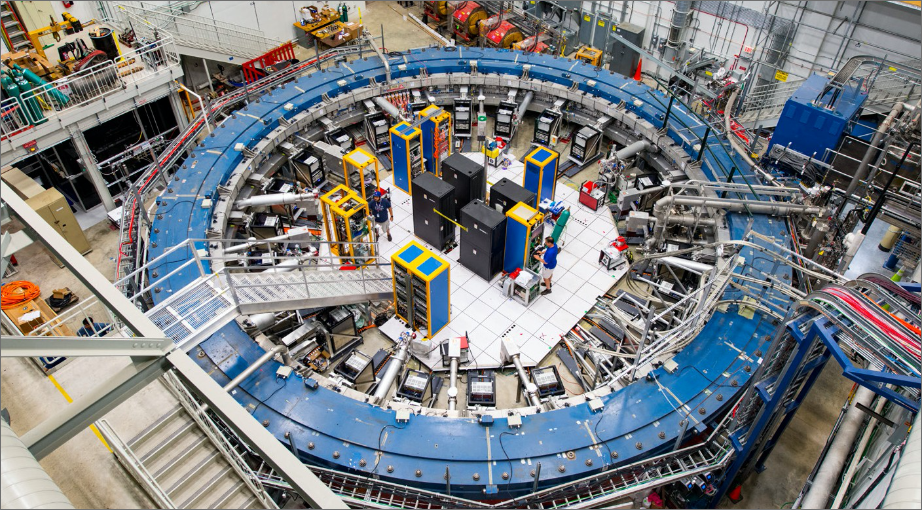
First results from Fermilab’s muon g-2 experiment hint at new physics, but the jury is still out on the final findings.
THE FIRST week of April was a momentous one for particle physicists. Scientists at the Fermi National Accelerator Laboratory in Illinois confirmed earlier findings that elementary particles, called muons, are more magnetic than expected, deviating ever so slightly from the expected theoretical prediction. This tiny discrepancy could be a sign of new physics, scientists say, perhaps an unknown force or new particle that subtly influences the muon’s magnetic behaviour.
Muons are subatomic particles very similar to electrons, but 200 times more massive and highly unstable. The Fermilab muon g-2 (read ‘g minus two’) experiment essentially measures, very precisely, how magnetic muons are. It is a long-awaited sequel to a measurement of the muon’s magnetic field done at Brookhaven National Laboratory, New York, in 2001. In both experiments, muons sped around a large, doughnut-shaped magnet (15 metres in diameter) in which the magnetic field makes them spin like tops. The more magnetic they are, the more they wobble or precess as they spin. The strength of its magnetic field or “magnetic moment” is measured by a quantity called the ‘g-factor’.
According to the Standard Model of particle physics, currently the most reliable theory describing the subatomic world, the muon’s magnetic moment is very close, but not equal, to 2. Twenty years ago, the Brookhaven experiment found that the difference, g-2, was slightly bigger than what theory indicated at the time. “It was the first tantalising clue that something was missing from the theoretical calculation,” says Priscilla Cushman, a member of the Brookhaven Muon g-2 team, now a professor at the University of Minnesota. Even though the 50-year-old Standard Model has successfully explained the fundamental particles and forces that make up the universe, it falls short in many ways. It does not explain dark matter and dark energy and does not account for neutrino masses or even gravity. We know that the Standard Model is not perfect and we have to modify it, but we don’t know how, says Cushman. Measurements that contradict theory are exciting because they hint at where the theory is lacking, she says.
The giant magnet was subsequently transported to Fermilab, where a more powerful muon beam and other technological tweaks would allow scientists to make a more precise and reliable measurement. The first run of data collection that culminated in the recent announcement took place in 2017 and 2018. Two more runs have been completed since, and a fourth run is under way. The goal is to improve the measurement’s precision by a factor of four.
For their part, theoretical physicists have been busy crunching the numbers while the muons race around the ring. In 2016, Aida El-Khadra, a particle theorist at the University of Illinois, worked with others to organise the Theory Initiative, an international consortium of 132 theoretical physicists who collaborated to nail down a theoretical Standard Model prediction of the g-factor. The magnetic moment of elementary particles is affected by fleeting “virtual” particles that they constantly emit and reabsorb. The contributions from all known particles are tallied up to arrive at the minute deviation of the muon’s magnetic moment. Last summer, the Theory Initiative reported the predicted value in a white paper and waited with bated breath to see if the experimental result would disagree with the calculations once again.
Given COVID-19 limitations, the results were unveiled to over 200 physicists on a private Zoom call in late February. The final value of g-2 depended on a number from a precise clock that was kept secret while scientists were gathering data and doing their analyses. This number was unveiled live on Zoom and plugged into the calculations. Members of the Fermilab team and University of Washington graduate students, Brynn MacCoy and Joshua Labounty were among those present. “I was holding my breath,” says MacCoy. “Seeing the final value of g-2 pop up on screen and agree so closely with the previous measurement was really exciting,” concurs Labounty.
WHAT THIS MEANS
This meant that the minuscule discrepancy between theory and experiment stands. “The difference is not large enough for us to claim that new physics has been discovered, but it is intriguing and heartening that it has remained,” says Thomas Teubner, a theorist at the University of Liverpool and a member of the Theory Initiative. While other claims that challenged the Standard Model have come to nought, the muon g-2 discrepancy continues to hold promise for new physics beyond the Standard Model, he says.
On the other hand, a Nature paper published on the same day as the Fermilab announcement has dampened the excitement for some. Calculations by a team of theorists called BMW reported a predicted value that is much closer to the experimentally measured magnetic moment. The approach used in the BMW calculations is relatively new, and different from the one adopted by the Theory Initiative. The latter is time-tested and has been refined over decades by many different groups. The BMW study must be taken seriously, but it is premature to take it at its face value, insists El-Khadra. Independent calculations from other teams that use the BMW approach are needed to confirm, or challenge, the robustness of that claim. As experimentalists continue to churn out ever-precise measurements, theorists have their work cut out as they weigh competing theories. “This is not the end of the journey by any means,” Teubner sums up. “I see these results as important milestones along the way.”
See also: The Standard Model of particle physics
Have a
story idea?
Tell us.
Do you have a recent research paper or an idea for a science/technology-themed article that you'd like to tell us about?
GET IN TOUCH