Flashes of mystery
-
- from Shaastra :: vol 03 issue 02 :: Mar 2024
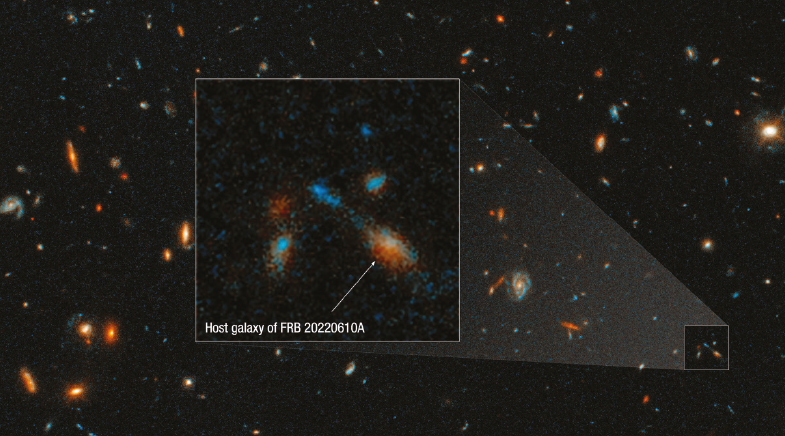
FRBs — high-powered, short-lived bursts of radio light — continue to baffle scientists. A new theory links them to and may help detect primordial black holes.
In 2007, Australian astronomers looking at archival data from the 64-metre Parkes telescope stumbled upon a mysterious flash of radio frequency light. It emanated from outside the Milky Way, lasted only some thousandths of a second, and was more powerful than the energy the Sun emitted in a year. The astronomers called it the Lorimer Burst, after one of the discoverers.
Scientists tended to dismiss the Lorimer Burst as an artefact, but, gradually, other such flashes were discovered. Over the years, nearly 1,000 such flashes have been detected with the development of sophisticated equipment.
On January 9, 2024, researchers in Northwestern University in the U.S. announced that one such flash — recorded on June 10, 2022, by the Australian Square Kilometre Array Pathfinder radio telescope and confirmed by the European Southern Observatory's Very Large Telescope in Chile — was the farthest in time so far. Using images from the Hubble Space Telescope, they concluded that this flash came from nearly 9 billion years ago – some 5 billion years after the Big Bang (bit.ly/farthest-burst).
Explanations for what causes the FRBs range from the straightforward to the exotic. Some invoke the presence of dark matter.
Astronomers, however, are nowhere near arriving at an explanation for this phenomenon. Called Fast Radio Bursts (FRBs), these flashes broadly fall into two categories — repeaters, which consist of a series of successive, short flashes of radio light; and non-repeaters, which only flash once, at least within the time of observation — as far as is known.
The repeaters do not pulse out identical flashes of light: the flashes seem to decrease in frequency, giving rise to what has whimsically been called the "sad trombone effect". It is not known whether the non-repeaters, too, will recur after an interval longer than the length of observation. Radio astronomers across the globe are now studying if the two types of FRBs have different explanations.
With data from the Canadian Hydrogen Intensity Mapping Experiment, observational astronomer Shriharsh Tendulkar, now at the Tata Institute of Fundamental Research, Pune, has located the origins of some of the 600-odd FRBs detected by it. The locations of the FRBs were expected to give a clue as to how they were created. However, knowing where they came from did not help. As the first few FRBs that Tendulkar's team found lay in dwarf galaxies, the members tried to find features of dwarf galaxies that could explain their formation. Later, however, FRBs were found in elliptical and spiral galaxies, too, although in small numbers (bit.ly/FRB-potential). So, these monster twinkles continued to baffle scientists.
MEASURING EXPANSION
Understanding and locating the FRBs may help in finding out, through an independent route, the value of the Hubble Constant, an astronomical quantity that helps measure the variation in the expansion of the universe. The universe is not only expanding, but points that are farther away from Earth appear to fly apart at a higher speed than those close by. The Hubble Constant is the rate at which the expansion velocity of the universe increases with distance (bit.ly/shaastra-hubble).
If the origin of the FRB is known, using the way it spreads through space — assessed by a quantity known as the Dispersion Measure — the Hubble Constant can be independently calculated. The recent discovery by the Northwestern University team would help in this endeavour.
However, it gets more complicated. This FRB appears to originate from a blob-like region where seven galaxies overlap. These galaxies appear to be strongly interacting with each other and could even be en route to a merger.
Explanations on what causes the FRBs range from the straightforward to the exotic. Some invoke the presence of dark matter, which has only been indirectly observed until now.
An interesting candidate among these theories has been put forth by research led by the Indian Institute of Technology (IIT) Bombay. This explanation wanders into gravitational waves, neutron stars and a special category of black holes that had hitherto only been hypothesised, namely, primordial black holes.
A NEW THEORY
As is now known, when colossal bodies — such as large black holes that can be tens of times the mass of the Sun or more — collide and merge, their union can cause its very fabric to shudder, making gravitational waves. These heavy bodies can consist of neutron stars, too, which are the end stages in the evolution of stars that are huge but not massive enough to form a black hole. Apart from this, there are primordial black holes that were hypothesised by Stephen Hawking in 1971. Unlike stellar-mass black holes, their masses can be low as compared to the Sun. These are believed to have been formed before stars were formed in the universe, so it is a mystery how they came into being. These have not been detected yet, but it is widely believed that they exist and influence other phenomena.
This theory, published in the Monthly Notices of The Royal Astronomical Society (bit.ly/FRB-explain), was proposed by S. Shankaranarayanan, Institute Chair Professor of Physics at IIT Bombay, and his collaborators – Ashu Kushwaha of IIT Bombay, and Sunil Malik of the Institute for Physics and Astronomy, Potsdam University, and the German Electron Synchrotron at Zeuthen.
According to the researchers, high-frequency gravitational waves arising from, say, a merger of primordial black holes can collide with and be absorbed by a neutron star that lies in their path as they travel towards the Earth. Any gravitational wave stretches and pulls spacetime as it passes. Thus, the wave absorbed by the neutron star distorts the spacetime in the star's interior. This contains intense magnetic fields. The pushing and pulling of spacetime containing the magnetic field causes an electric field to be born. This is similar to the action of Ampère’s law, according to which a changing magnetic field can give birth to a coupled electric field.
When there is a match in their frequencies, the electric and magnetic fields can form an electromagnetic wave that leaves the neutron star. These electromagnetic waves, the three researchers propose, will be the high-frequency FRBs observed. Just like a radio transforms electric fields into sound waves, the neutron star transforms these gravitational waves travelling through it into radio-frequency light (or electromagnetic waves).
The effect of gravitational waves interacting with a magnetic field to produce electromagnetic waves, or light, is called the Gertsenshtein-Zel’dovich (GZ) effect. The three researchers invoke this effect to get the desired outcome: a burst of electromagnetic wave showers, which are the fast radio bursts in this context.
Gravitational wave detectors — LIGO in the U.S. and Virgo in Italy — detect gravitational waves in the frequency range of 100 Hertz to 1,000 Hertz. This network has detected many mergers of black hole pairs or black hole-neutron star pairs. However, it is believed that gravitational waves of frequencies outside this range exist.
The mass of the merging bodies varies inversely to the frequency of the gravitational wave emitted by the merger. So, gravitational waves of higher frequency are generated by mergers from black holes with masses that are much smaller than the ones detected so far.
BACKGROUND BUZZ
Adding an exciting dimension to this story, a collaboration of four groups — the NANOGrav across North America; the European Pulsar Timing Array; the Parkes Pulsar Timing Array in Australia; and the Chinese Pulsar Timing Array — announced in June 2023 that there was a random background buzz of gravitational waves from the cosmos, quite like rainwater on a pond. The collaboration estimated that the frequency of gravitational waves emitted would be in the nanohertz range.
Into this pixelated half-image of the spectrum of gravitational waves, enter the postulated primordial black holes. Since the masses of primordial black holes can be smaller, if pairs of these merge, the reasoning is that the gravitational waves that they cause will be of high frequency, ranging from mega to gigahertz.
To establish this theory, Shankaranarayanan and his group have to prove that the process is indeed given by the GZ effect. They are now working on defining signatures for this effect that can be seen by observing the fast radio bursts.
Have a
story idea?
Tell us.
Do you have a recent research paper or an idea for a science/technology-themed article that you'd like to tell us about?
GET IN TOUCH