The Standard Model of particle physics
-
- from Shaastra :: vol 01 edition 01 :: May - Jun 2021
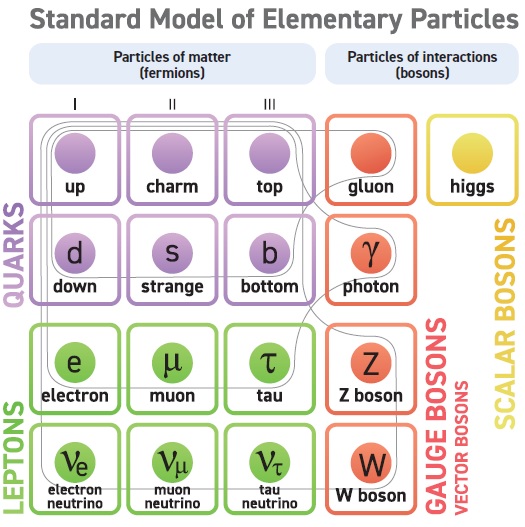
Tracing the evolution of the theoretical framework that laid the foundation for a broader understanding of matter. It seems ripe for a ‘rejig’.
SOMETIME IN the 5th century BCE, Greek philosopher Leucippus and his student Democritus said that all matter was composed of atoms. There is some controversy about who really first thought of the idea – Leucippus or Democritus – but the student, rather than the master, is now largely credited with first proposing that matter consisted of hard, indivisible particles called atoms. Democritus did not know what atoms were made of but posited that matter became indivisible at some point. We then reach the smallest constituent of matter, the atom. It was more philosophy than science.
Aristotle reigned supreme in Europe till the Renaissance, which meant that Democritus and his ideas were forgotten for 18 centuries. The first modern proponent of the atomic theory was John Dalton, a British chemist, who noticed that substances reacted with each other in definite, whole-number ratios. For example, hydrogen and oxygen combined in ratios of 2:1 to produce water. It was a good hint of the existence of atoms, but there was no direct evidence till Einstein formulated the theory of Brownian motion and Jean Perrin observed it in 1908. Brownian motion is the constant jiggling of particles in a fluid, caused by random motion of atoms.
However, scientists knew that atoms were not indivisible – even before their existence was proved early in the 20th century. In 1897, British physicist J.J. Thomson observed a negatively charge particle during an experiment to discover the real nature of cathode rays. These rays are produced when a high voltage is applied between the ends of a small tube from which most of the gas has been emptied. Thomson found that these rays consisted of a stream of negatively charged particles that he called corpuscles, but they were later renamed as electrons. It was obvious to scientists that the electrons came from within the atoms and hence the atom was not indivisible.
The Standard Model explains a large number of natural phenomena, but it is not a complete theory
‘PLUM PUDDING’ VS ‘SOLAR SYSTEM’
Thomson constructed a ‘plum pudding’ model of the atom in 1904, which looked rather like a breakfast muffin filled with raisins. The negative electrons and the positive particles – the atom was neutral, and so there had to be positive particles to balance the charge – were distributed uniformly in the plum pudding model. This idea didn’t last for very long. Ernest Rutherford, a New Zealand physicist at Cambridge University, found in 1911 that the positive charges were concentrated in the middle of the atom and not spread around the atom.
Rutherford had bombarded thin gold foils with positively charged alpha particles generated from radioactive materials. Most of the particles went through the foil easily, but some bounced right back at the source. The alpha particle, which we now know consists of two protons and two neutrons, would bounce back only if it encountered a strong and concentrated positive charge. Because most of the particles went through the foil, the Rutherford experiment showed that the atom was largely empty space. Rutherford changed the plum pudding model into one based on the solar system, with the positive charge concentrated in the middle and the electron revolving around it.
In 1932, James Chadwick, another British physicist, discovered the neutron.
By the early 1930s, physicists thought they knew about all the particles required to build the atom – and, by extension, all matter in the universe. The neutrons and protons made the core of the atom, and the electrons moved around the core. Quantum mechanics had forced scientists by then to abandon the solar system analogy in favour of the more fuzzy idea of orbitals, where the electron did not have precise orbits around the nucleus of the atom. It was still a simple picture, consisting of a few elementary particles.
Till 1932, the only other known particle – apart from the electron, the neutron and the proton – was the photon, the particle of light. It wasn’t a particle like the electron or a proton, as it was not a building block of matter. Before the end of the 1920s, British physicist Paul Dirac had predicted the existence of a positive electron; it was discovered in 1932. In his Nobel lecture the next year – it took only one year for the Nobel Committee to award Dirac the prize – Dirac proposed an antiproton as well, with the same mass but opposite charge as the proton. The antiproton took much longer to be discovered. Nature seemed to have a short-lived anti-particle for every particle. But, for no obvious reason, matter particles dominated the universe.
THE MYSTERIOUS MUON
In 1937, American physicists Carl Anderson and Seth Neddermeyer discovered the muon. It was an unusual particle that seemed to have no business to exist. What purpose did the muon serve in the universe? It was like a heavy electron, with a similar negative charge, that was so burdened by its weight that it disintegrated into an electron and another particle very quickly. This unknown particle was thought to be the neutrino, but is now known to be its antimatter version, the antineutrino. The neutrino was discovered in 1956.
The list of elementary particles increased in the 1960 and 1970s to beyond 60. Nature was proving to be more complex than scientists had imagined. In 1964, American physicist Murray Gell-Mann theorised that the proton consisted of smaller particles called quarks, the first of which was discovered in 1968 at Stanford. Soon after the discovery of quarks, physicists started building a model that explained all matter and their interactions. They classified the particles into two main groups, the particles of matter and particles of interactions, and formulated elaborate rules about what each particle can and cannot do. This model came to be known as the Standard Model of particle physics.
The particles of matter in the model were the electron, the muon, the tau, three kinds of neutrinos and six different quarks. The particles of interactions are exchanged during an interaction, creating a force between the matter particles. The photon is exchanged for the electromagnetic force, the gluon for the strong nuclear force, and the Z and W bosons for the weak nuclear force. These forces drove events in the universe. The strong nuclear force held the protons and neutrons together in the nucleus. The electromagnetic force made atoms possible. The weak force was responsible for radioactive decay. The fourth fundamental force, gravity, is supposed to be mediated by the graviton, but it has not been directly detected yet.
THE HIGGS BOSON
There was one serious problem, however, with the model. No one knew how the elementary particles got their masses. British physicist Peter Higgs wrote in a paper in 1964 – it was initially rejected by journals – that a field present throughout the universe resists the motion of particles, and it is this resistance that is felt as the mass of the particle. The more a particle feels the field, the more massive the particle becomes. There are some particles – the photon – that do not feel the Higgs field and hence do not have mass. The Higgs field can produce a particle under certain conditions. This particle, called the Higgs boson, was discovered by scientists at the Large Hadron Collider in 2012. It was one of the greatest triumphs of physics. The Higgs mechanism was used by Abdus Salam and Steven Weinberg in 1967 to develop a unified electroweak theory that combined the weak force and the electromagnetic force.
The Higgs mechanism was used by Abdus Salam and Steven Weinberg in 1967 to develop a unified electroweak theory that combined the weak force and the electromagnetic force.
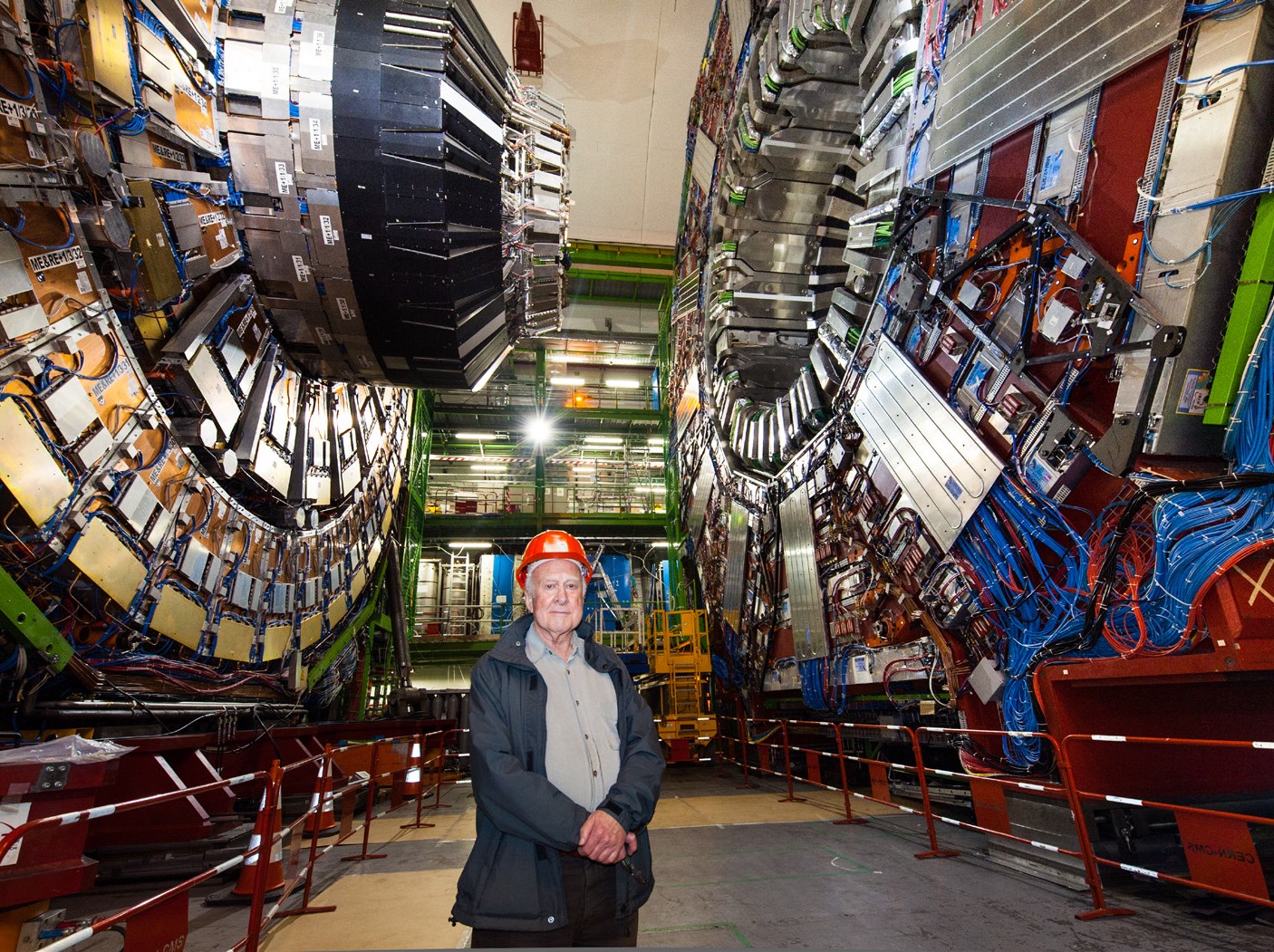
The electroweak force is an important part of the Standard Model. After Gell-Mann proposed the quark, physicists found them mysterious. The protons and neutrons were composed of three quarks, with different flavours combining in different ways in each, but no single quark could be isolated. On the other hand, quarks also moved freely within the proton at a very short distance. Three American scientists – David Gross, Frank Wilzcek and David Politzer – deduced that the strong force was weak at very short distances inside the proton but became stronger as they floated apart. At some distances, it would be so strong that the quarks can never come off each other. So it seems that a single quark can never be isolated.
UNANSWERED QUESTIONS
The Standard Model explains a large number of natural phenomena but it is not a complete theory. It does not reconcile two major theories of physics: quantum mechanics and Einstein’s theory of general relativity. It does not account for dark matter, the mysterious substance that is known to exist throughout the universe. It does not explain why neutrinos have mass. It does not explain why matter – and not antimatter – dominates in the universe. Occasionally, physicists encounter anomalies of particle behaviour that cannot be explained by the equations of the Standard Model. The muon experiment at Fermilab was one such anomaly. The Standard Model seems to be ripe for rejig. To be more precise, it is ready for a significant extension.
Have a
story idea?
Tell us.
Do you have a recent research paper or an idea for a science/technology-themed article that you'd like to tell us about?
GET IN TOUCH