Looking beyond the double helix
-
- from Shaastra :: vol 01 edition 02 :: Jul - Aug 2021
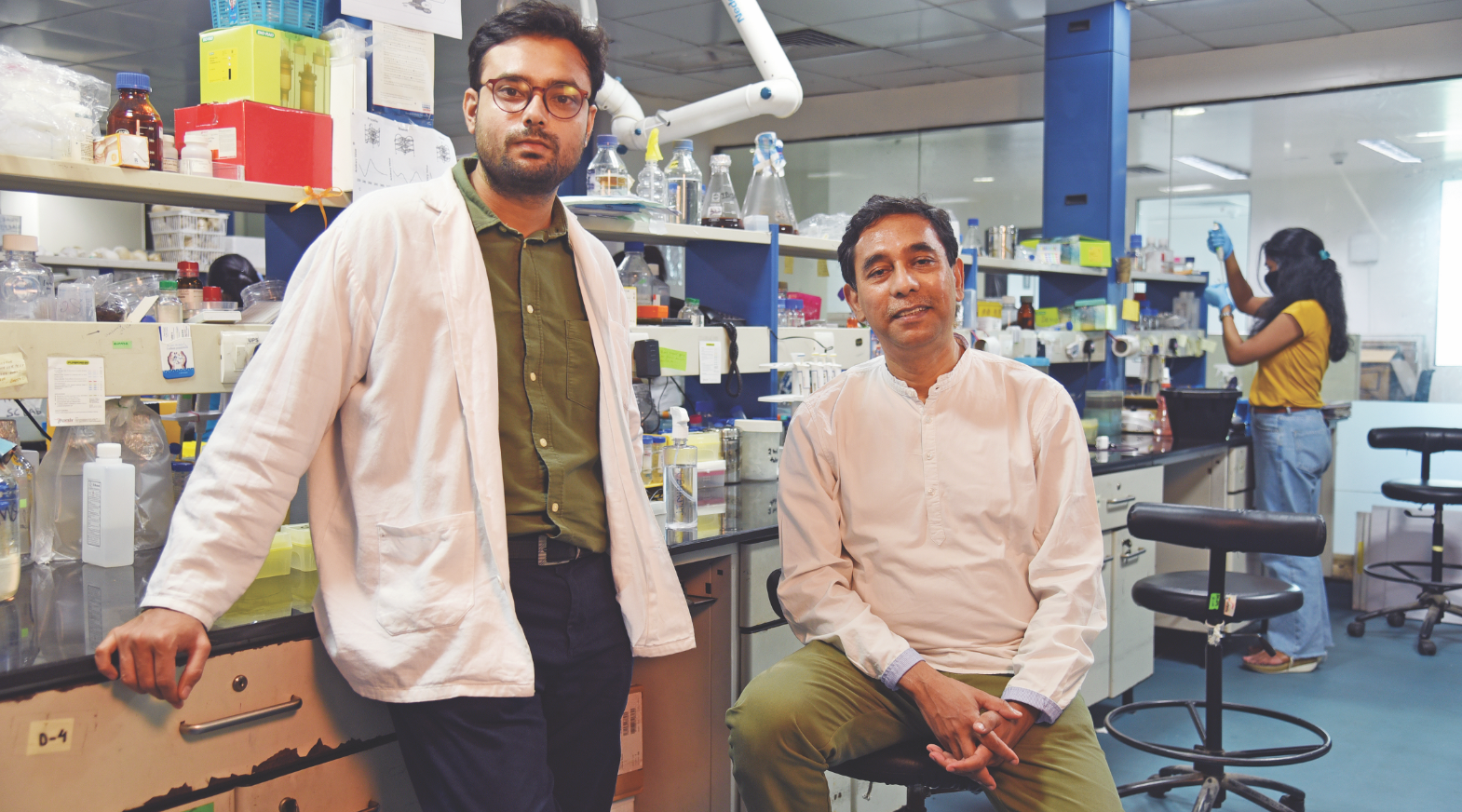
A recent study provides novel insights into potential therapeutic opportunities to target cancer.
In 2013, American and German scientists discovered two mutations that occurred in 80% of brain cancers, 71% of skin cancers, and to a lesser degree in bladder and liver cancers. The mutations were in the telomerase enzyme that plays a role in maintaining telomeres - the end regions of chromosomes. The telomere acts like a protective cap of the chromosome, but it becomes shorter and shorter with each cell division. The cell dies when the telomere reaches a certain length, which is a mechanism for old cells to make way for new ones. Cancerous cells, through mutations, seem to hijack this machinery and divide forever.
The findings of that research study, published in Science journal, had got the attention of scientists around the world who were working on telomeres. Among them was Shantanu Chowdhury at the Institute of Genomics and Integrative Biology (IGIB) in Delhi. Chowdhury's research centred around the enzyme telomerase, which is necessary in telomere synthesis. How the cell regulates this enzyme is poorly understood; however, scientists know that its production is shut down in normal adult cells but reactivated in cancer cells. Chowdhury and others had been working on the problem.
The mutation discovered in 2013 occurred in a region of DNA that was rich in a chemical base called guanine. The DNA in this guanine-rich region folds into four stacked sheets, which are together called G-quadruplexes (G4). The mutations occurred in the G4 sequence and disrupted its quadruple sheet structure, which somehow led to telomerase-reactivation in some cancers. "This information kept haunting me. I kept thinking about the core mechanism and interesting avenues where we can exploit telomerase-specific G4 for therapeutic benefits," says Chowdhury.
Chowdhury had been interested in G4 since 2002, when he joined IGIB as a young scientist. It was soon after the human genome was sequenced, and technologies had become available to sequence large genomes quickly. His group analysed several genome sequences to discover that G4s occur in promoters - on-off switches - of bacterial genomes, and are conserved in around 700 gene promoters of human, mouse and rat genomes. This year, in a Cell Reports paper, Chowdhury's research group cracked the mechanism of how the G4 region controlled the expression of telomerase, and hence held the key to some cancers.
An important player in this game is a protein called TRF2. Ananda Kishore Mukherjee, a PhD student in Chowdhury's laboratory, observed that TRF2 can bind to telomerase, the enzyme that is involved in protecting telomeres. Mukherjee had analysed that TRF2 binds at around 20,000 sites in the human genome and regulates activity of many gene promoters that harboured G4 sequences. This suggested that TRF2 is not only confined to telomeres but can park itself in many G4-rich sequences, some of which can regulate telomerase expression in the cell.
So, Chowdhury's group showed that TRF2 binding to the G4 shut down the expression of telomerase in adult cells. Some mutations inhibit the ability of the G4 to fold into stacked sheets, and therefore disrupt its structure. The disrupted structure does not let TRF2 to bind, which means that the expression of telomerase is not shut down. With the telomerase being produced constantly, the cell does not die, but it continues to divide. The actual mechanism would be much more nuanced, but the main culprits seem to have been caught.
Chowdhury's PhD student Shalu Sharma confirmed through experiments that TRF2 binds to the telomerase promoter - the genetic switch of telomerase synthesis - and shuts down telomerase expression. "Studies by Sharma in the Chowdhury lab provide evidence that TRF2 may have functions in addition to binding to telomeric DNA," says Jerry Shay, telomere biologist and Distinguished Professor of Cell Biology at the University of Texas Southwestern Medical Center, in an email communication. "Importantly, this study provides novel insights into potential therapeutic opportunities to target cancer," adds Shay.
Logic suggests that one way to treat such cancers is by shutting down telomerase activity and thereby letting the telomeres shorten in length once again. Shutting telomerase activity requires the restoration of the G4 sheet structure lost due to mutations. The genetic mutations in the DNA cannot be reversed, but scientists hoped to discover small molecules that could restore the original structure of the G4.
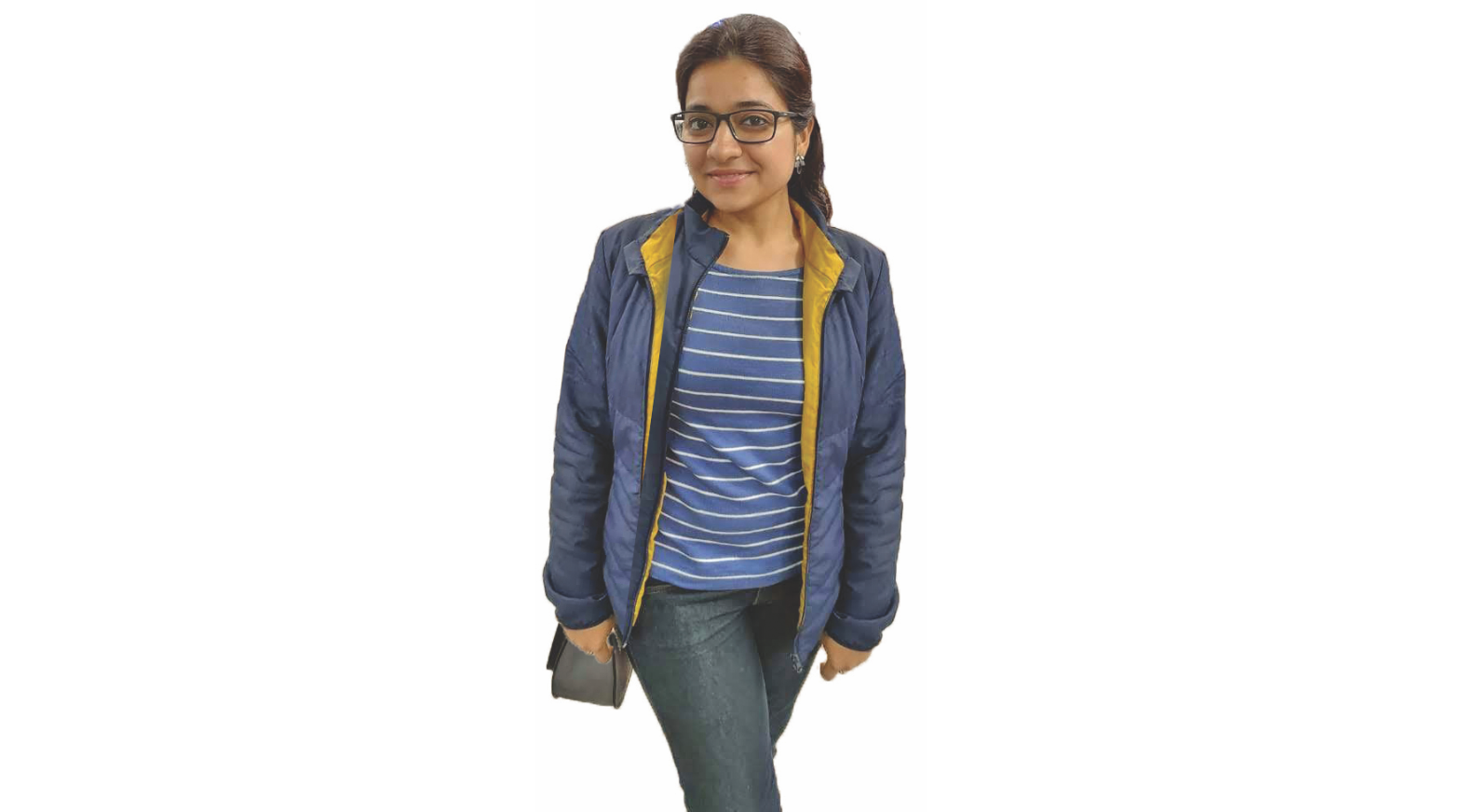
The importance of G4 in gene regulation suggests their significance as drug targets in a wide variety of diseases, especially cancers and infections. They offer an advantage as drug targets over their protein counterparts. Most anti-cancer drugs work by jamming the function of proteins that are overexpressed in cancer cells, but all proteins may not bind all drugs owing to constraints posed by either their size or their structure. There is a specific fit for the protein-drug duo. Certain cancer cells can become resistant to drugs used in clinical practice, and thus pose requirements for novel molecules.
Binding molecules circumvent this problem as they target the G4 DNA structure to shut the synthesis of wrong proteins. Quarfloxin was the first G4 targeting anticancer molecule and it has progressed to phase II clinical trials against several types of cancers, and other approved drugs are under study for G4 binding properties.
Jyotirmayee Dash, chemical biologist and professor at the Indian Association for the Cultivation of Science (IACS) in Kolkata, designs molecules that can bind to G4. Unlike Chowdhury, who studies G4 in live cells, Dash looks at how small molecules can interact with G4 in a solution. She had earlier worked with Shankar Balasubramanian, a Cambridge professor who had proved the existence of G4 in human cells. Not all G-rich sequences would form a quadruplex structure inside the cell, and such studies are important to figure out how a specific sequence can fold into an actual quadruplex structure. In a recent piece of research published in Chemistry Europe journal, Dash and her students synthesised a novel molecule that can specifically bind to G4 in a cancer gene promoter and suppress its expression in blood cancer cells.
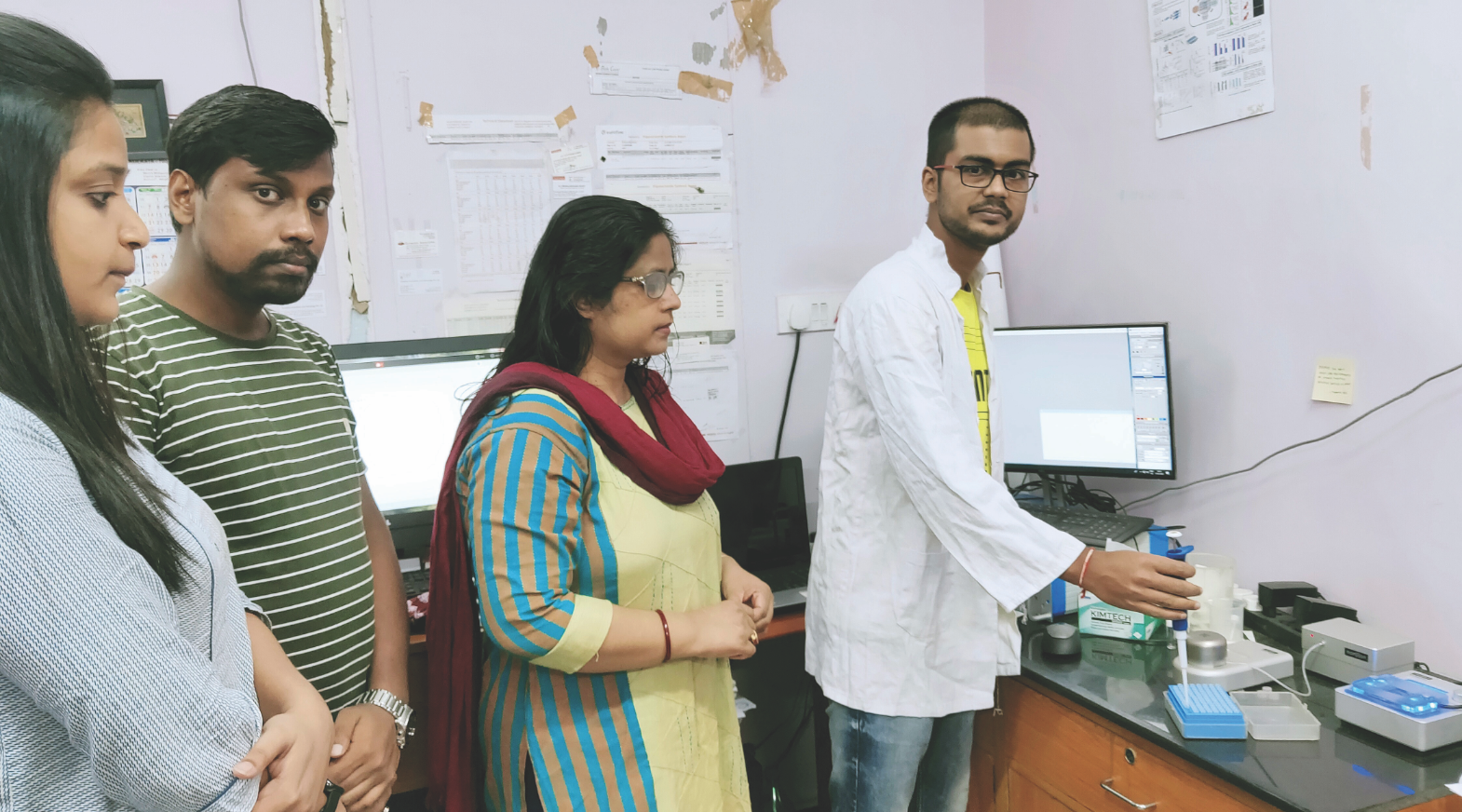
G4 has been shown to exist in a wide variety of organisms, including bacteria and viruses. Vivekanandan Perumal at the Indian Institute of Technology Delhi studies G4 in viruses, as a prelude to investigating ways of developing treatments against viral infections. Perumal got interested in the topic after listening to a talk by Chowdhury. “While listening to Shantanu, I thought that G4 secondary structures must be present in viral genomes,” says Perumal. The area has not been studied well, and Perumal aims to explore G4 in viruses.
Perumal investigated the hepatitis B virus, which has ten subtypes that differ in the way they cause infection. Can these differences be explained by G4-based mechanisms? Perumal worked on a virus with a small genome, and found that it had only one G4 sequence not seen anywhere else in the genome. He showed that a disruption of a single G4 sequence affected every step of viral replication. He also found that the genome of herpes virus has dense G4 sequences.
The G4 can turn out to be important for solving three big healthcare problems: cancer, viral infections and bacterial infections.
In both herpes and hepatitis B viruses, G4 sequences were in regions responsible for viral infection. G4 evolution shows interesting patterns in viruses and their hosts. G4 are abundant in vertebrate viruses and very scarce in invertebrate viruses. "If you look at genomes of viruses that infect humans, they are rich in G4 such as herpesviruses," says Perumal. "On the contrary, if you analyse G4 in an invertebrate genome such as a mosquito, it has little G4. Likewise, viruses that infect mosquitoes lack G4 sequences." It is also a suggestion that G4 are good targets for antiviral drugs.
Meanwhile, Dash is investigating G4 sequences as targets for anti-bacterials. So, the G4 can turn out to be important for solving three big healthcare problems: cancer, viral infections and bacterial infections.
Have a
story idea?
Tell us.
Do you have a recent research paper or an idea for a science/technology-themed article that you'd like to tell us about?
GET IN TOUCH