A new organelle on the block
-
- from Shaastra :: vol 03 issue 04 :: May 2024
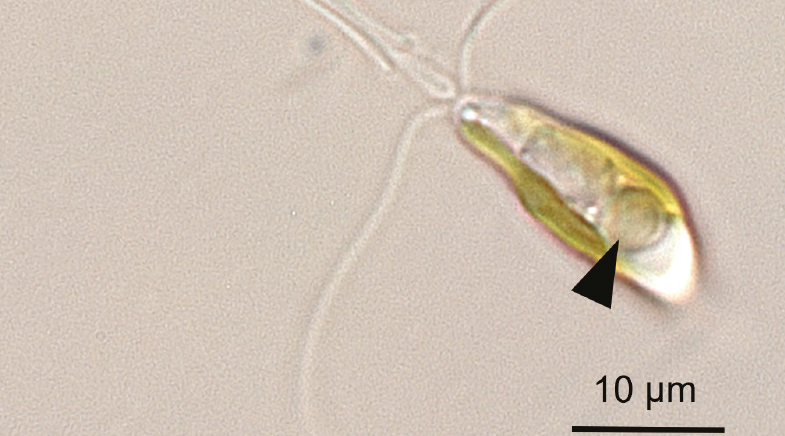
Scientists find a nitrogen-fixing cyanobacterium living as an organelle inside a marine alga.
For decades, marine biologist Jonathan Zehr from the University of California (UC), Santa Cruz, has been interested in a tiny bacterium from the Pacific Ocean. It started in 1998, when he found a short DNA sequence from what was then an unknown nitrogen-fixing cyanobacterium. The research group at UC Santa Cruz named this UCYN-A, short for Candidatus Atelocyanobacterium thalassa. This bacterium had a reduced genome and a consequent inability to fix carbon or photosynthesise. The researchers guessed that it must be dependent on another organism to make up for this deficiency. Such interdependent relationships are common in nature and called symbiosis.
Around the same time, Kyoko Hagino, a palaeontologist from Kochi University, Japan, was trying to grow a marine alga in her lab and facing many challenges with it. The alga, Braarudosphaera bigelowii, is also the symbiotic partner, or the host organism, for UCYN-A. It took Hagino well over a decade and 300 sampling expeditions to grow B. bigelowii in her lab. But once she achieved this milestone, it unlocked the possibility of probing the alga deeply. Since UCYN-A lived inside the alga, researchers also had the chance to get to know it better and, more importantly, understand the relationship between UCYN-A and its host B. bigelowii.
The bacterium UCYN-A does not merely share a symbiotic relationship with its host alga; it has evolved into an organelle of the alga.
SYMBIOSIS AND EVOLUTION
Zehr's lab at UC Santa Cruz studies how microorganisms control the availability of nitrogen, and was especially interested in UCYN-A. Now, in papers published in Cell (bit.ly/endosymbiont) and Science (bit.ly/nitroplast), with two different but overlapping sets of authors, the research group has established that the bacterium UCYN-A does not merely share a symbiotic relationship with its host alga B. bigelowii. Rather, it has evolved into an organelle of the alga. It has no independent existence but helps the single-celled host in fixing nitrogen – pretty much as the mitochondria generate energy in cells.
Such evolution from an 'endosymbiont' – a symbiont living inside the host cell – to an 'organelle' – a sub-cellular structure taking care of a specific job – is a rare phenomenon. Since the evolution of life, this has happened only a few times. The oldest example of this is the mitochondrion. About 2 billion years ago, mitochondria evolved from purple non-sulphur bacteria that migrated into another organism as an endosymbiont. Even today, some traces of these primitive bacteria are visible in our mitochondria as mitochondrial ribosomes and tRNAs (transfer ribonucleic acid). Though this presumably happened in one pair of species, now the mitochondrion is part of most eukaryotic cells (cells that have a nucleus). Another example is the chloroplast, which was captured some 1.5 billion years ago and helps in photosynthesis.
There are varying degrees of symbiosis in plants – one extreme case being endosymbiosis, where a bacterium can live inside the host plant and exchange benefits while retaining its own individuality. Despite living inside the host, this relationship is sometimes reversible, sometimes not. Still, one can imagine a thought experiment where the endosymbiont is taken forcibly out of the host cell and allowed to adapt, and it can do that in order to pursue an independent existence once again. In cases where it cannot survive, the endosymbiotic relationship has gone past the point of no return, and the two are inextricably entwined into one entity.
This is the juncture at which the research group finds the alga B. bigelowii and the bacterium UCYN-A. The group has discovered that the bacterium UCYN-A is no longer just an endosymbiont. It has become so dependent on the host that it is now a part of it. It is an organelle that fixes nitrogen, and scientists are calling it 'nitroplast'.
FIXING THE NITROGEN
Nitrogen is essential to plant life, but plants do not have the capacity to absorb it directly from the atmosphere. They need it "fixed" in the form of ammonia, and nitrogen-fixing is an important task that bacteria do to help plants. To help in this process, they live in close connection with the plant as symbionts. An example of this is the rhizobia bacteria that live in the root nodules of leguminous plants such as peas or beans. For their part, the leguminous plants fix atmospheric carbon during photosynthesis, which they supply to the bacteria to energise them. This is at the heart of the symbiosis between the bacteria and the plant. In the system being studied now, the nitroplast is said to have been integrated less than one million years ago, as proposed by Hagino in 2013 (bit.ly/Hagino-PLOS).
"It is a snapshot of the evolutionary mechanism in action," says Satyajit Rath, Professor of Biology at the Indian Institute of Science Education and Research (IISER) Pune, who was not involved in the work.
ORGANELLE FORMATION
The researchers offer three sets of observations as evidence of the organellogenesis. First, the size of the nitroplast inside the B. bigelowii cell is conserved with respect to the size of the cell. Second, B. bigelowii codes for proteins that are exclusively meant for use by the nitroplast. Third, the host cell controls the division of the nitroplast, and it is co-ordinated with its own division.
Despite years of study, being able to show this came as a surprise to the scientists. "Seeing unexpected patterns in hard-won data is what scientists live for," says Tyler Coale, lead author of the Science paper from Zehr's lab, who contributed to the proteomics study. According to Coale, the prime motivation of the study was to understand ocean biochemistry, and the cycling of carbon and nitrogen in the world's oceans. Nitrogen fixation in the marine environment can fuel ocean ecosystems from the base of the food web, but the organisms that perform this function are largely understudied. "I don't think any of us expected this research to become of interest to such a wide array of scientists," he says. "It's a feeling of excitement followed immediately by the need to dig deeper, explain and substantiate the finding."
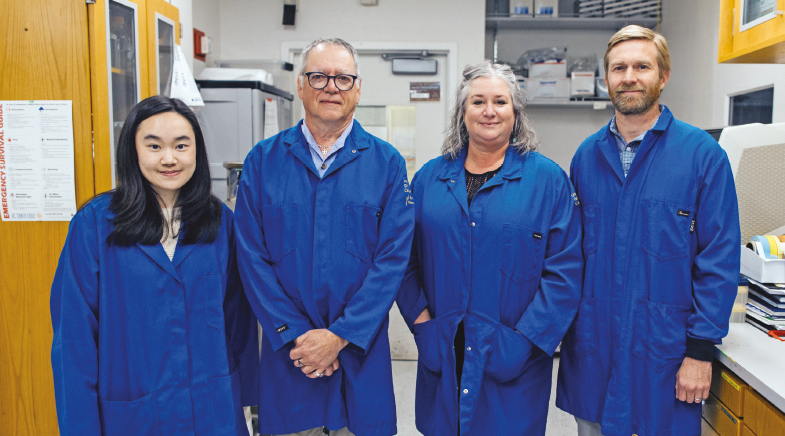
One of the key tools used in imaging the algal cells was soft X-ray tomography, a tool to capture the micrometre-sized alga in three-dimensional images. In recent years, novel imaging techniques have been investigated to provide insights into cellular structures that accurately reflect the structural changes necessary for cells to carry out specific roles or functions. "The utilisation of soft X-ray tomography, pioneered by Carolyn Larabell, has proven particularly valuable in analysing these alterations in cell architecture," says Valentina Loconte from the University of California, San Francisco, and Lawrence Berkeley National Laboratory, who performed the acquisition, analysis and interpretation of the soft X-ray tomographic data. Loconte was a post-doc with Larabell and came into this project when Larabell and Zehr started collaborating. "When Jon Zehr presented the challenge of understanding the relationship between UCYN-A and B. bigelowii, we were extremely excited to explore a fundamental question in biology such as organellogenesis," says Loconte.
"It was fascinating to observe the highly synchronised division process of the alga and UCYN-A..."– Valentina Loconte
When she initially observed the coordinated division from the soft X-ray tomography data, she was uncertain whether she was witnessing cell replication. However, upon reconstructing the data, it became evident that the cell was undergoing cell division, showing that the newly divided UCYN-A was present in the dividing algae. "Successfully reconstructing the life cycle of B. bigelowii was thrilling," says Loconte. "It was fascinating to observe the highly synchronised division process of the alga and UCYN-A, along with the systematic reorganisation of (the) cell's architecture."
Mukund Thattai, cell biologist at the National Centre for Biological Sciences, Bengaluru, has no hesitation in calling the nitroplast an organelle of B. bigelowii. As UCYN-A has lost so many genes, it is unlikely to be able to lead a life of its own even if uprooted from the host in a thought experiment, he says. Where Thattai differs from the authors of the papers is on why such transitions are extremely rare. "They are hard because any co-operative mechanism is hard to sustain over long periods," he says. For instance, in B. bigelowii, the relationship can be traced back to a million years. If in the intervening years the alga had gone extinct, this relationship would probably not have been discovered. "I feel, if the symbiosis lasts long enough, it will certainly grow tightly regulated. But it has to last: that's the hard part," says Thattai.
Have a
story idea?
Tell us.
Do you have a recent research paper or an idea for a science/technology-themed article that you'd like to tell us about?
GET IN TOUCH