Smothering the flames of instability
-
- from Shaastra :: vol 01 edition 01 :: May - Jun 2021
Scientists at IIT Madras channel an intuitive understanding of complex system dynamics to solve the problem of ‘combustion instability’.
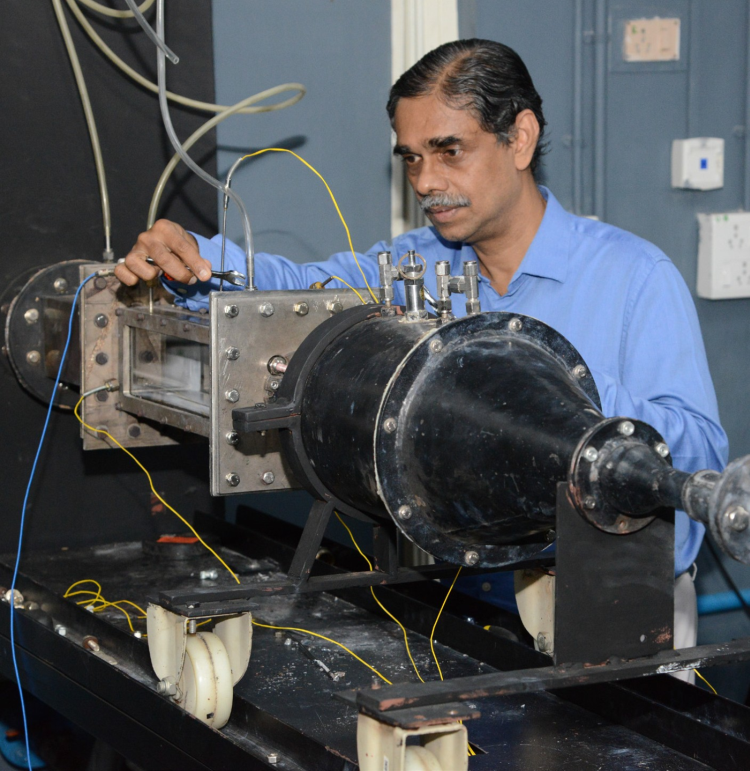
ONE NIGHT in August 2013, R.I. Sujith, a professor of aerospace engineering at IIT Madras, uncharacteristically awoke just before 3 a.m. in a state of intense excitement. He had just had an epiphany of sorts – although the scientist in him would probably say he experienced a ‘Eureka!’ moment.
The ‘revelation’ he had related to one of the most vexing problems in his field of study: how to stop small fluctuations in flames from becoming large and destroying gas turbines and rocket engines.
A month earlier, Sujith, who researches combustion instability, especially in turbines and rocket engines, had attended a conference in Hyderabad. The conference was on non-linear dynamics, an area of research for mathematicians and theoretical physicists. One dealt with hardcore engineering, the other with abstract mathematics. In that sense, it was an unusual conference for an engineer to attend, but Sujith had sensed a connection between these two fields and wanted to learn non-linear dynamics and complex networks.
The ‘combustion instability’ problem that Sujith had been pondering was as old as the subject itself. Engineers who designed the rockets for the Apollo Mission had got around it by conducting 3,200 full-scale tests that taught them how to design engines where flames were not self-destructive. But they had merely sidestepped the problem: they did not understand the mechanism of flame instabilities. Five decades after the Apollo Mission, engineers were still trying to understand how flames become unstable in turbines and rocket engines.
That August morning in 2013, the possible reasons for combustion instabilities came to Sujith in a flash. “It stayed for a few minutes… the feeling that I knew everything,” recalls Sujith. He immediately wrote down what he could remember.
FROM INTUITION TO EQUATION
Sujith had intuitively felt the connection between complex networks and instabilities in combustion, but the details would take a long time to work out. Specifically, he understood that complex networks – for instance, airline routes, the World Wide Web, the food chain – share features with flames. However, he had to translate his intuitive sense into a set of equations that could be solved. Developing these equations, which would describe the behaviour of combustion using the mathematics of complex networks, was a long-haul project. “Knowing is not understanding, being able to calculate everything,” he says. “I am still at it.”
Combustion instability affects gas turbines and jet and rocket engines, and Sujith’s team’s work used a complex network approach to address it.
In 2014, Sujith began to use the mathematics of complex networks in his research. His paper, published in 2015 in the Journal of Fluid Mechanics, was the first paper in thermoacoustics or combustion that used complex networks.. The field is now growing very fast, with scientists and engineers using complex systems mathematics to understand combustion dynamics. In the past five years, Sujith’s group has produced several insights that could help turbines and jet engines improve their performance.
Combustion instability can affect turbines and jet and rocket engines – with disastrous consequences. Jet engines avoid the problem by severely restricting the conditions of flight. In other realms, combustion instability can cause turbines to be shut down, entailing revenue loss for utility companies.
In other words, this was an important problem to solve. People’s lives depended on it, and so did business fortunes.
Sujith’s special research interest was thermoacoustic instability, a phenomenon where the sound produced by the engines effectively enhances the flames destructively. While this problem has been around for a while, engineers largely took a reductionist approach to get rid of the noise. Sujith’s team, however, decided to look at the issue holistically using a complex network approach. “I decided that this part-by-part approach cannot be applied to solving the thermoacoustic problem as the oscillations generated in the system form a complex network and influence each other,” says Sujith.
A complex network has a large number of closely interacting parts that produce effects not easily predictable by studying its components. In fact, a complex system follows the metaphysical dictum that the whole is more than the sum of its parts. Complex networks are all around us: the neurons in our brain connected by synapses, billions of computers and mobiles connected through the World Wide Web, and living organisms at various levels linked through the food webs. Individual elements (or nodes) in the networks are connected to each other. Just over two decades ago, scientists made an interesting discovery about these networks: some nodes have only a few connections while others have a large number of connections.
This fact seemed counter-intuitive in the beginning. Why should some nodes in a network have more connections than the others? It turned out that any network that keeps growing can exhibit such a feature when there is a preferential attachment to one node. A preferential attachment means that some web pages generate more interest than others, or some companies are able to produce more partnerships, and so on. Such networks are called scale-free networks, in contrast to a random network where no node dominates. The World Wide Web was supposed to be a random network – until experiments showed otherwise, that a few web pages have a large number of connections. Sujith’s first paper using complex networks found that combustion noise was scale-free.
In complex networks, individual nodes are connected to each other in such a way that the whole is more than the sum of its parts.
In simple terms, this meant that the vortices that form while burning have no preference for any size. Sujith and his students converted the data that they got from combustion into complex networks by using specific algorithms, and showed that it exhibits scale-free characteristics. It also gave Sujith a method to predict when it is about to lose its scale-free nature. It often means trouble for the engine or turbine.
During experiments in combustion, Sujith had noticed that the faces of his students became tense before the onset of thermoacoustic instability, when the sound produced while burning interacts with the flame to produce vibrations and noise. Such vibrations can produce cracks in the wall of the engines or cause unsteady burning in the engines. The students, who perform a large number of experiments in the lab, could somehow sense the onset of instability before it happened. Now, with the complex network principles, Sujith found an intermediate stage before the full instability happened.
Sujith’s team found that upcoming instability in the combustion system can be predicted by the nature of oscillations. When the system is stable, the oscillations are small in amplitude and aperiodic; then, in the intermittent stage, there are bursts of large-amplitude oscillations; and finally, in the unstable stage, the oscillations are periodic but with large amplitude. This movement from small-amplitude to large-amplitude periodic oscillation is actually the movement of a system from a complex network to a regular network: a network where every node has a similar number of connections and hence the system has no large hubs which can influence the whole network.
PUTTING OUT THE FIRE
Sujith’s team was therefore able to successfully predict the upcoming disaster; its next aim was to get rid of this instability. They decided to again apply the complex network approach to mitigate the upcoming instability.
“In Kalari martial arts, if specific pressure points of an opponent’s body are poked, the rival is unable to move any body part. This is because the particular body part that has been poked is like a hub and has connections with various other vital body parts or function. We decided to find such a point or hub in the system which if perturbed can restore back the system,” explains Sujith.
Sujith’s team found a way to identify regions that were critical to controlling instability. Microjects injected in those regions can reduce the amplitude of the oscillations at the intermediate stage to a level that is comparable to what is observed during the state of stable operation. This injection in the critical region can stop the turbine’s path from stability to instability and prevent blasts and other disasters.
In parallel, Sujith also found another feature of complex systems during his combustion: chaos or lack of order. Although counter-intuitive, nature is chaotic and yet stable. Lack of chaos often means trouble. A healthy heart is chaotic, and loss of this feature is a sign of impending problems. A well-burning flame is also chaotic, and loss of this feature is also a sign of trouble, just like loss of scale-free behaviour.
Chaos and scale-free behaviour are now established as important features of flames that are stable. But scientists are yet to relate them to each other. That is where Sujith wants to take his research in the future.
Have a
story idea?
Tell us.
Do you have a recent research paper or an idea for a science/technology-themed article that you'd like to tell us about?
GET IN TOUCH