When bacteria go brrr…
-
- from Shaastra :: vol 03 issue 09 :: Oct 2024
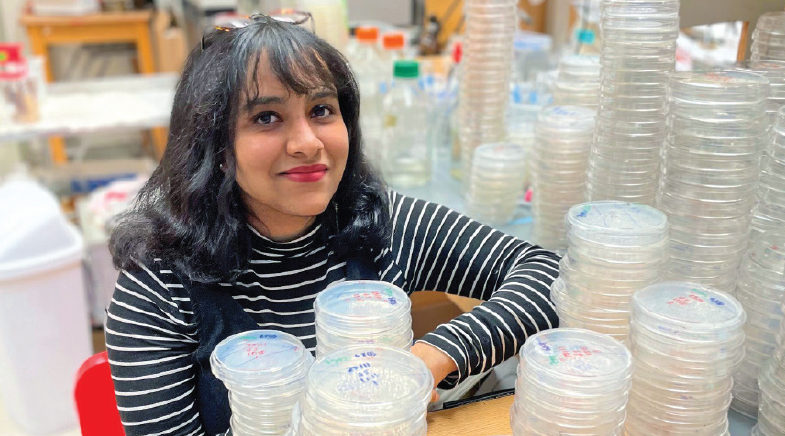
Cyanobacteria use their body clocks to sense oncoming seasons. Can this finding be used to mitigate algal blooms?
Biologist Carl H. Johnson has a 'Post-it' stuck on his office door at Vanderbilt University in Tennessee. Progress, it says, is made by young researchers who conduct experiments that their bosses say will never work. So, when his PhD student Maria Luísa Jabbur saw that he was sceptical about her thesis proposal, she was disappointed but didn't give up.
"I was a little discouraged, but the feeling didn't last very long because shortly after expressing scepticism, Carl told me that I should try the idea out anyway," says Jabbur, now with the John Innes Centre in the U.K.
Jabbur wanted to see if microorganisms such as cyanobacteria — tiny organisms with a generation time of just a few hours — sensed the advent of seasons and prepared themselves for the changes. Her research led her to discover that being exposed to short or winter-like days promoted cold resistance in cyanobacteria.
Her study focused on how the tiny microbes sensed and measured differences in the lengths of day and night — unequal durations of exposure to light and darkness in a lab. While a closely related element, the circadian clock mechanism, had been well studied in cyanobacteria — Johnson himself had done so for decades — not much was known about their response to light periods, or photoperiodism. Could photoperiodism be some kind of an environmental signal for cyanobacteria, she wondered.
Jabbur and her collaborators demonstrated through her experiments (bit.ly/bacteria-season) how cyanobacteria could gauge the changes in day lengths by measuring the duration of light and thereby sense oncoming cold weather and gear up for it. These tiny organisms, which do not even have a nucleus, could code and convey multigenerational signals.
HELPING THE EARTH GROW
Small in size and bluish-green in colour, cyanobacteria get energy from photosynthesis. They are commonly known as blue-green algae, though they are not really algae. Some species of cyanobacteria existed even under very primitive conditions on Earth when its atmosphere had little oxygen. Over billions of years and continuous photosynthesis, these tiny autotrophs transformed the Earth's atmosphere. Due to their photosynthesis, the Earth grew rich in oxygen, and this subsequently led to the formation of single-celled organisms, which, in turn, captured simpler organisms, leading to the evolution of eukaryotes or organisms with a nucleus.
Johnson, the Cornelius Vanderbilt Chair & Professor, Biological Sciences, and Professor, Molecular Physiology and Biophysics, has been studying cyanobacteria for over 30 years. He was a part of the groups of researchers who showed that cyanobacteria had circadian clocks (bit.ly/cyanobacteria-circadian); found three genes that coded for and constituted this clock (bit.ly/gene-cluster); and demonstrated that these microorganisms had an evolutionary advantage because they possessed the circadian clock (bit.ly/bacteria-fitness). Even to someone like Johnson, with years of experience in the subject, it seemed far-fetched that these short-lived prokaryotes could sense and respond to seasons that change over months.
Jabbur persisted in her research. In the first set of experiments, the cyanobacteria were divided into two groups. One was kept in an environment where they experienced short hours of daylight and long hours of darkness to mimic what happens in winter. The length of daylight progressively decreased over the cycles. The other group was kept in an environment where they experienced equal stretches of light and darkness. Following a few such cycles, each group was subjected to a sudden, cold environment — a "cold shock". The research aimed to see who survived the cold shock.
The difference was so stark that one could tell there was an effect just by looking at the number of bacterial colonies on each plate, even before quantifying it: cyanobacteria that had been subjected to shorter daylight regimes survived the cold shock, while those experiencing equally spaced "days and nights" did not. The cyanobacteria had learnt from the shortening days that they were going to experience cold weather, and they had geared up for it, as the group discovered later, by changing the chemical composition, lipids, etc., of their cell membranes to be more protective against the cold.
There was a lot of guesswork involved, and Jabbur cites as an example that most of the results shown on the paper are after she gave the cyanobacteria eight cycles of light/dark. "Later on, we learned that the number of cycles matters, and they need about 4-6 cycles to develop the response." But she had chosen eight cycles simply because she wanted to give the short-day cells enough time to grow. "Looking back, there was just a lot of luck involved," she says.
SHORT LIVES, LONG GAINS
Sheeba Vasu, a chronobiologist from the Jawaharlal Nehru Centre for Advanced Scientific Research, Bengaluru, who works with Drosophila, finds it striking that even organisms that live for only a few hours can gain an evolutionary advantage if they can prepare for what happens over multiple generations. "This is the first experimental demonstration that even in a cyanobacterium, the machinery that has evolved for daily timekeeping is also being used for seasonal timekeeping," Vasu says.
Jabbur has also verified that the circadian clock was indeed involved in measuring the differences in the length of day and night, which appeared to be the key to the bacteria developing cold resistance. She did this by studying bacteria in which the three circadian clock genes had been removed. Such modified bacteria did not show any difference in their response to cold shock, whether or not they had been subjected to differential lighting.
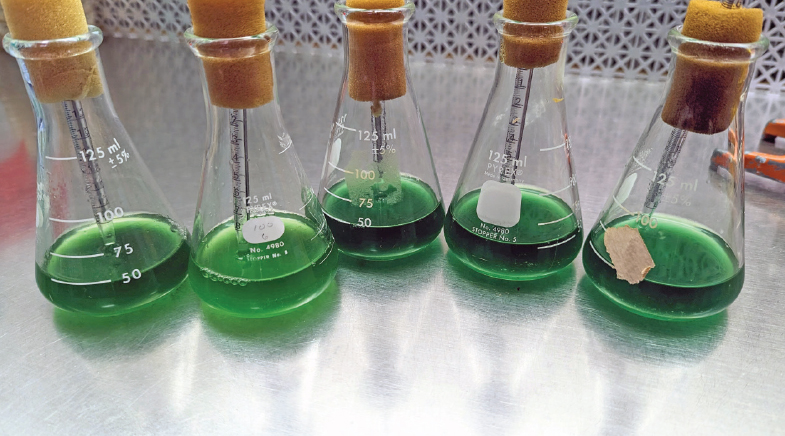
The research has thrown up a lot of questions for Jabbur. "I want to know what genes are involved, what pathways, and particularly how the cells are passing the seasonal information along (to) the next generations," she says. Her interest is in studying the bacteria's response to climate change.
The bacteria experienced different types of stresses; short days were stressful because the microbes had to survive long nights when they could not synthesise energy.
In particular, cyanobacteria are known to form algal blooms. Algal blooms are a seasonal, intense proliferation of cyanobacteria and some other species in waterbodies, so fierce that the colour of the water itself changes. Algal blooms are sometimes toxic. They harm the local fish, and this has a substantial economic impact. They can also indirectly cause ill health in those who consume the fish. This becomes uncontrollable with climate change and unpredictable algal blooms. Jabbur believes that if this seasonal response can be understood better, it may be mitigated.
Climate change is known to affect photoperiodic responses such as bird migration and flowering, but is hard to study in higher organisms as they take longer to grow and reproduce. Cyanobacteria, on the other hand, form a good model organism for studying the evolution of photoperiodic responses under climate change.
STRESSFUL DAYS
Johnson, meanwhile, is interested in the evolutionary aspects of the timing devices in cyanobacteria. His idea stems from the fact that during the experiment, the researchers noted that there were different types of stresses that the bacteria experienced during short and long days. Short days were stressful because the industrious microbes had to survive long nights when they could not synthesise energy by photosynthesis. On the other hand, long days were stressful because, despite gaining energy, there could be damaging ultraviolet light. Jabbur's experiments showed that these stress pathways evolved in the long-versus-short-days pattern.
"I found this interesting because we assume that evolutionarily, it is the circadian clock that evolved first and later got enlisted to measure day length and then to measure photoperiodism," Johnson says. "But this work of Luísa's, looking at gene expression pathways, has me thinking about this differently."
He explains that if in organisms such as cyanobacteria, which need light to survive, these different stress responses get triggered on exposure to long days as opposed to short days, it is probably more urgent to evolve a timer for the day duration. Therefore, the first timer that had evolved was likely to be a simple hourglass-like timer, and not a circadian clock that flipped at every cycle.
The research leads to new thoughts on the evolution of time-sensing mechanisms. That long-lived organisms gear up for seasonal changes is known. The fact that cyanobacteria can sense the coming of winter may help scientists further understand photoperiodic responses to climate change.
Have a
story idea?
Tell us.
Do you have a recent research paper or an idea for a science/technology-themed article that you'd like to tell us about?
GET IN TOUCH