The cosmic rush
-
- from Shaastra :: vol 03 issue 06 :: Jul 2024
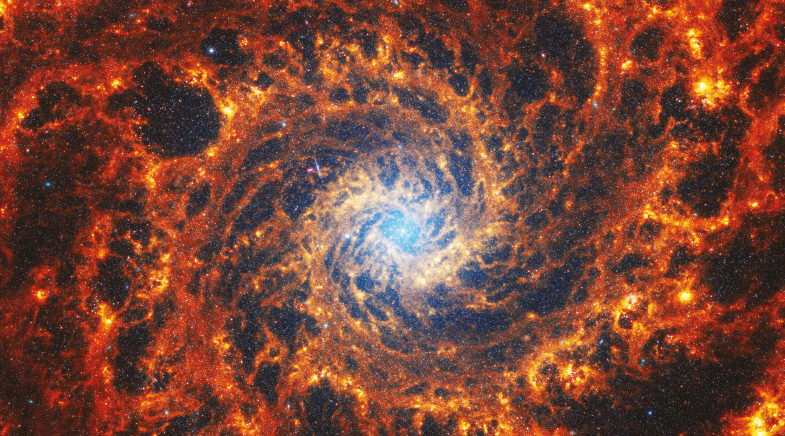
A series of new space missions is expected to answer some of the deepest questions in cosmology.
Subir Sarkar felt that things were rapidly coming to a head in his field of research. He needed to organise an international conference in cosmology.
Trained first as a particle physicist, Sarkar had been feeling uncomfortable for a while about his current field of research. Both particle physics and cosmology have reigning champions called the standard models, written differently to distinguish them from each other. The Standard Model of particle physics was a well-proven theory. The standard cosmological model, on the other hand, was what the name originally meant: a model. “Particle physicists get up every day thinking about ways of breaking the Standard Model,” says Sarkar, Professor Emeritus in the Department of Physics at the University of Oxford, “because that is how we make progress. But in cosmology, the standard cosmological model is becoming a religion.”
The standard cosmological model is a mathematical description of how things are in the universe, how they got here and how they are evolving. It posits that the universe began with the Big Bang 13.8 billion years ago and has been expanding ever since. A relic of the hot early universe was discovered in 1964, as radiation that fills the universe everywhere, a remnant of the first light that escaped the hot dense state about 400 million years after the Big Bang. Towards the end of the 1990s, physicists found the first evidence to suggest that the universe’s expansion was accelerating. Physicists also extrapolated from data they gathered from telescopes: that the universe had six times more matter than what we can see, that something called dark energy was driving the acceleration. They also assumed that the universe looked the same in all directions.
In the past decade, several questions have arisen about these ideas, driving cosmologists roughly into two camps. The smaller group, of which Sarkar is a leading representative, thinks that the current model is outdated and needs more physical evidence for adoption. The larger group, which believes in the validity of the standard cosmological model, says that the model may need to be merely tweaked to fit the new observations. “I say cosmology is not complete,” says James Peebles, Albert Einstein Professor of Science, Emeritus, at Princeton University and winner of the 2019 Nobel Prize in Physics (The universe according to James Peebles). “It means there is a better theory that would do better to resolve the anomalies that we have. It’s a good bet also that when this new theory is found and the anomalies are resolved, there will be new anomalies.”
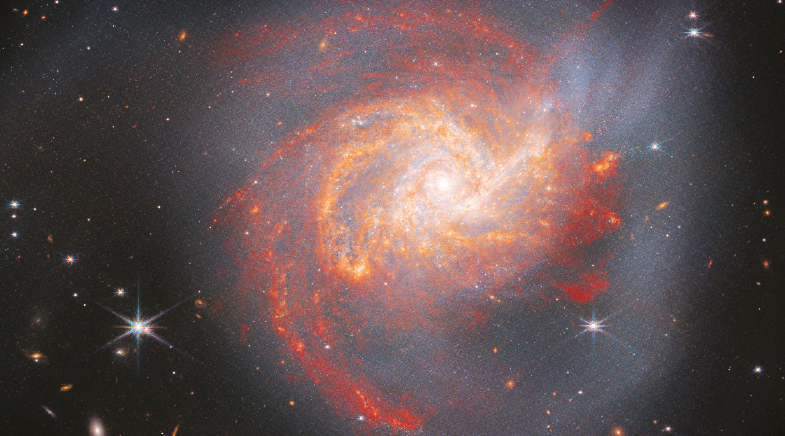
The meeting, convened in London in April by Sarkar and other colleagues along with The Royal Society, was called ‘Challenging the standard cosmological model’. It was intended to debate these differences and move the model forward – or find a candidate to replace it. It was critical for cosmologists to resolve the differences. Tied to a correct model of cosmology were big questions about the composition, evolution and ultimate fate of the universe. As the meeting closed and the differences remained, cosmologists turned their attention to a series of missions to be launched over the next few years, including those that are already collecting voluminous data about the universe. Astronomers expect such astronomical observations to push their field further into the golden age it is supposed to have entered recently.
In December 2023, Mayall, a ground telescope at the Kitt Peak National Observatory, Arizona, released the first results of a survey of nearly six million galaxies, which constituted roughly 20% of the sky. Called the Dark Energy Spectroscopic Instrument (DESI), it was built to look at the large-scale structure of the universe over long periods of time. Its announcement suggested that dark energy was changing over time. It is now analysing data from a few more millions of galaxies, and hopes to survey around 30 million galaxies within a few years. Cosmologists have rarely had access to such rich data about the universe over time.
In July 2023, the European Space Agency (ESA) and the National Aeronautics and Space Administration (NASA) launched a probe called Euclid, with the purpose of making a three-dimensional map of the dark universe – which astronomers do not see through their optical telescopes. Situated near Lagrangian Point 2 – where it can remain in orbit while requiring less energy to do so – on the far side of the Sun-Earth line, Euclid will eventually survey billions of galaxies out to ten billion light years away and therefore ten billion years back in time, specifically focusing on the dark matter and dark energy that is reckoned to be present throughout the universe.
There are even more precision missions being developed. The Nancy Grace Roman Space Telescope, to be launched by NASA in 2027, will survey the sky for two kinds of data: accurate measurements of supernovae and variations in the density of normal matter over large distances. It will have a sensitive camera and calibration capabilities not achieved so far in any instrument, thereby providing exceptionally accurate data about sources. Complementing its capabilities is the Vera C. Rubin Observatory, being constructed in Chile and starting operations in January 2025. With a 3-gigapixel resolution, it has the largest digital camera ever built, which can capture images from tens of thousands of square degrees every few days, one square degree being about four times the size of a full moon. Both telescopes have been named after distinguished American astronomers.
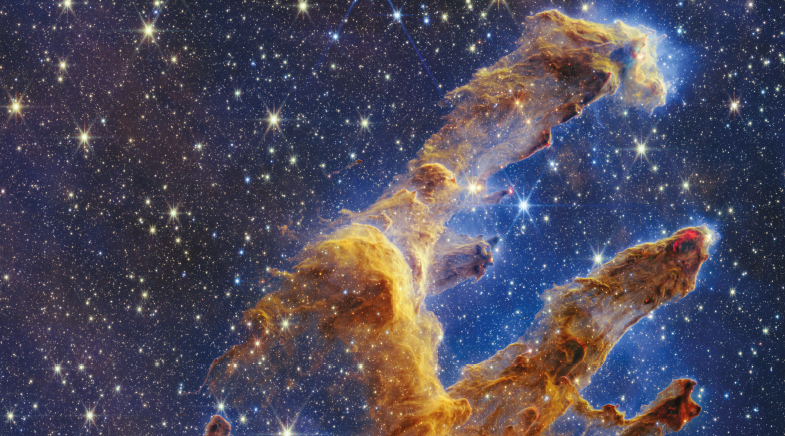
In India, the Indian Institute of Technology (IIT) Bombay is driving the construction of the twin satellite Daksha. This pair will observe the sky for light released when neutron stars merge, a violent event that produces gravitational waves as well. Measuring the light from their mergers helps cosmologists get an independent measure of the expansion of the universe.
A consortium of Indian scientists, called CMB Bharat, has proposed a mission to study the imprint of the earliest gravitational waves on the cosmic background radiation, called the B mode. This is a subtle effect that is beyond the capabilities of any instrument today. “This is often considered the holy grail of cosmology,” says L. Sriramkumar, Professor of Physics at IIT Madras, who is part of the consortium. “If we measure the primordial B mode, it will tell us the energy scale of the epoch of inflation.”
India is already part of the Square Kilometre Array, a set of radio telescopes that can estimate dark energy to a great accuracy. All these missions together provide data at such formidable range and depth that cosmologists expect some of their deepest questions to be answered soon. “The Nancy Grace Roman Space Telescope is designed more than anything else to give precision and accuracy to cosmological measurements,” says Dominic Benford, Program Scientist for the Nancy Grace Roman Space Telescope. “That power from statistics and systematics will allow us to measure the universe and answer this question once and for all.”
This question is the precise expansion rate of the universe, one of the big unsolved puzzles in cosmology.
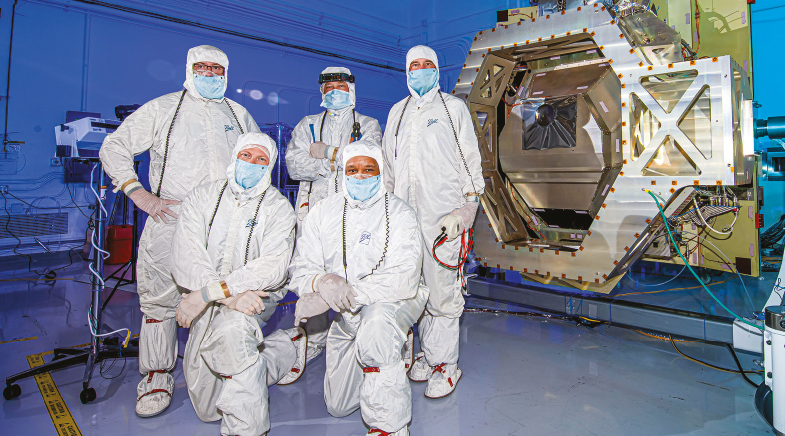
BUILD-UP OF TENSIONS
In 1916, Albert Einstein developed a set of equations that described gravity as a property of space-time and not as a force. Since gravity played an important role in the evolution of stars and galaxies and all other structures, the correct solution of these equations would provide insights into the history and future of the universe. So, along with these equations, Einstein started a new era in cosmology.
When Einstein developed his equations, he didn’t know that the universe was expanding. His equations told him that space-time had to expand or contract, but he couldn’t come to terms with an expanding universe. He introduced a term, called the cosmological constant, as a trick to counter an expanding universe. Later, when data showed that the universe was expanding, he removed that term, calling it his greatest blunder. He didn’t know that the cosmological constant would reappear in a different garb in later models of the universe.
In 1929, the American astronomer Edwin Hubble found the definitive proof for expansion of the universe when he noticed that galaxies were all flying away from each other. The farther the galaxy was from Earth, the faster it moved away, a phenomenon consistent with the expansion of space everywhere. Others had come to the same conclusion before Hubble, notably Alexander Friedmann from Russia and Georges Lemaître from Belgium. By the end of the century, two independent observations found that the expansion rate itself was accelerating. No one knew the reason for this expansion, and its cause was named with the nondescript term dark energy. The easiest way to describe dark energy in mathematical terms was through Einstein’s cosmological constant.
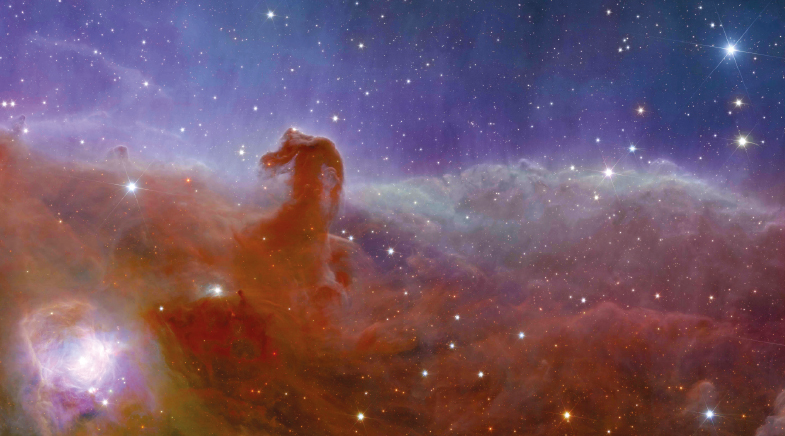
Over time, as scientists started measuring more precisely the present rate of expansion (called the Hubble constant, or H0), different methods gave different results. They were not too different and yet differed enough to be taken seriously. One method was a simulation starting with data from the early universe, and the other was going backward from data from the present. “It is like building a train track from two ends,” says Benford, “and when they come together, they don’t quite meet.” Cosmologists named this problem as the Hubble tension. The discrepancy was roughly 10% and was a cause for serious debate at the Royal Society conference.
The Planck Scientific Collaboration, a set of scientists who contributed to the Planck mission of the ESA, calculated the Hubble constant forward from the early universe data. The Planck mission was live from 2009 to 2013 and measured the background radiation from the early universe with great accuracy. The Cosmic Microwave Background (CMB) radiation can be measured from as early as when the universe was 400 million years old, when its temperature was about 3000 Kelvin, which is roughly 2727°Celsius. Now the temperature of CMB radiation is about 2.7 Kelvin.
Several questions have arisen about the standard cosmological model, driving cosmologists roughly into two camps.
This radiation is supposed to come at a uniform temperature from all directions, but scientists have measured patches where the temperatures are slightly different. Scientists know that matter and energy were clumpy at the beginning of the universe and by how much. By calculating forward from those values and comparing them with density variations now, they can calculate the expansion rate of the universe. The Planck collaboration team got a Hubble constant value of 67.5 km/sec/megaparsec, a megaparsec being 3.2 million light years. It means that the expansion rate of space increases by 67.5 km/sec every 3.2 million light years from Earth.
The Hubble constant can also be calculated by looking at present-day objects and then going backwards in time. Astronomers measure distances usually from looking at how bright objects appear from Earth and then correlating with their real brightness. This technique is useful only when the intrinsic brightness of an object can be calculated; two such sources with known brightness are a type of supernovae and a type of star called Cepheid variables. Similarly, once the colour of an object is known, astronomers can also calculate how fast it is moving by measuring how much the colour shifts towards the red end of the spectrum. Using these methods and looking at nearby objects and faraway objects, some astronomers calculated the Hubble constant to be 73 km/sec/megaparsec. The best-known such result came from a team led by Adam Riess at Johns Hopkins University, a Nobel Prize winner and one of the scientists who figured out that the expansion of the universe is accelerating. This team used data from the Hubble Space Telescope, and was part of a scientific collaboration called SH0ES, an acronym for Supernova, H0, for the Equation of State for dark energy.
The James Webb Space Telescope (JWST) now provides astronomers with much clearer images compared to the Hubble Space Telescope. Wendy Freedman, Professor of Astronomy and Astrophysics at The University of Chicago, used the JWST to calculate the Hubble constant, but using three different types of stars to make the estimate. Her value, which was presented at the Royal Society conference, was 69.8 km/sec/megaparsec, which was smack in the middle of the other two values. Freedman believes that it is consistent, assuming experimental errors, with the standard cosmological model. “There is no requirement for new physics based on these new data,” Freedman said in an e-mail response to Shaastra.
Not everyone is convinced. Riess himself believes that Hubble data was too voluminous to be ignored, and that the JWST would take time to build such a repository. A Nature article from April 2024 quotes him as saying, “The Hubble Space Telescope has collected a mountain of data over several decades, including four separate and one direct calibration” of the Hubble constant.
NOT THE SAME EVERYWHERE
The standard cosmological model is a simple mathematical description of the universe using just six parameters that have to be experimentally determined. It describes a universe that began expanding after the Big Bang, with the expansion rate increasing through some unknown means. In the model, about 26% of the universe is dark matter, which no one has seen, and 69% is dark energy that is supposed to push space apart faster and faster. Physicists assume that the universe looks the same everywhere, provided we sample over long distances. This homogeneity, called the cosmological principle, is another way of saying that matter and energy distribution have no preferred direction when observed from suitably large scales. Recent data question the assumption.
The first instance of an anisotropy, which is what physicists call a property that varies based on the direction we look, in the cosmic background radiation was noticed in the 1960s. Peebles explained this anisotropy is because of the movement of the Earth and its neighbours through this radiation. This movement seemed to account for the radiation anisotropy, which was one-thousandth of the expected value one side of the Earth. However, the anisotropy in matter distribution could not be explained away so easily.
A test for this was devised in 1984 by George Ellis, a physicist from South Africa, and John Baldwin, a radio astronomer from Cambridge. To actually perform the test, a million stars had to be catalogued.
Sarkar and his collaborators used a type of objects called quasars to perform the test. Ashok Singal of Physical Research Laboratory in Ahmedabad had tried it earlier but could not muster enough objects. Sarkar used a catalogue created by Nathan Secrest, an astronomer at the U.S. Naval Observatory. It was an all-sky sample of 1.36 million quasars observed by NASA’s Wide-field Infrared Survey Explorer (WISE) mission. The group found that not only was there an anisotropy, it was about twice as much as expected.
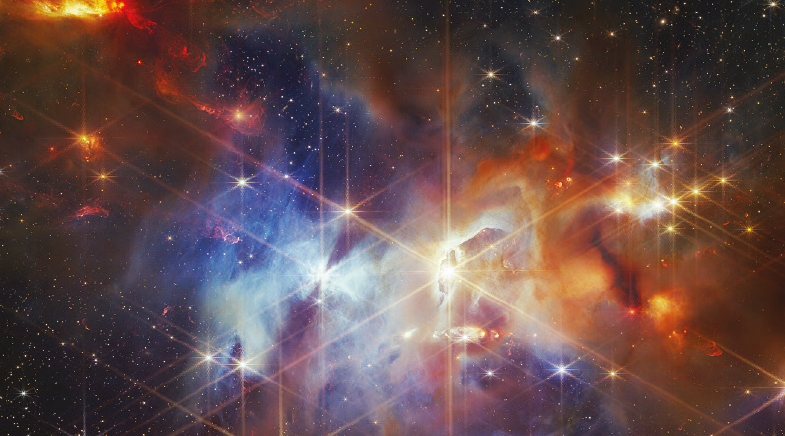
The standard cosmological model assumes that every point is equivalent to every other point. According to Sarkar, it is because scientists assume that the universe is seen to be made up of 68% dark energy and that the expansion rate is accelerating. “The foundation of this is the assumption that the universe is homogeneous and isotropic at large scales,” he says. In the ten years, astronomers have got enough data to test this assumption, that the universe is isotropic – has no preferred direction – in the reference frame that the background radiation is isotropic. “To our surprise we find it isn’t so.”
Peebles, on the other hand, feels that there is no assumption in standard cosmological model that the universe is homogeneous. “It only violates (the) cosmological principle if you assume that the principle says (that) at large scales the universe is exactly homogeneous,” he says. In other words, small amounts of clumps in the early universe can be enlarged over time into large differences in homogeneity at the largest scales. Does that explain enough the observed inhomogeneities? Future missions may give the answer.
This ostensible anisotropy has recently been supplemented by another mystery: the universe around the Milky Way – a part that is one billion light years across – is moving in one direction at an average velocity of 400 km/s. This is according to results published in July 2023 by Richard Watkins, Professor of Physics at Willamette University in Oregon. Some astronomers assumed the presence of a large body, called the great attractor, to explain this flow, but such a body has not been found. A few months after Watkins and his colleagues published their paper, Yehuda Hoffman, Professor of Physics at The Hebrew University of Jerusalem, used the same data but different techniques to come to the same conclusions. While Watkins reported a high level of confidence in the results, Hoffman reported much lower levels of confidence, insufficient to contradict the standard cosmological model but enough to recognise that the Earth is present in an unusual part of the universe.
As more precise data become available, astronomers are noticing more unusual phenomena. Last year, for example, DESI telescope data hinted that dark energy density was changing. So far, the constancy of dark energy – represented by Einstein’s cosmological constant – has itself been a mystery. If a box of gas expands, for example, the density of the gas reduces as it fills a larger volume. If space expands, how does dark energy keep up with the expansion and remain constant? The DESI result is an indication that dark energy may not indeed remain constant. “All of us are very excited,” says Shadab Alam, a faculty member at the Tata Institute of Fundamental Research (TIFR) Mumbai and a member of the DESI collaboration. “But we have to be careful this is not a statement of confidence.” More data may raise the statistical significance of this finding.
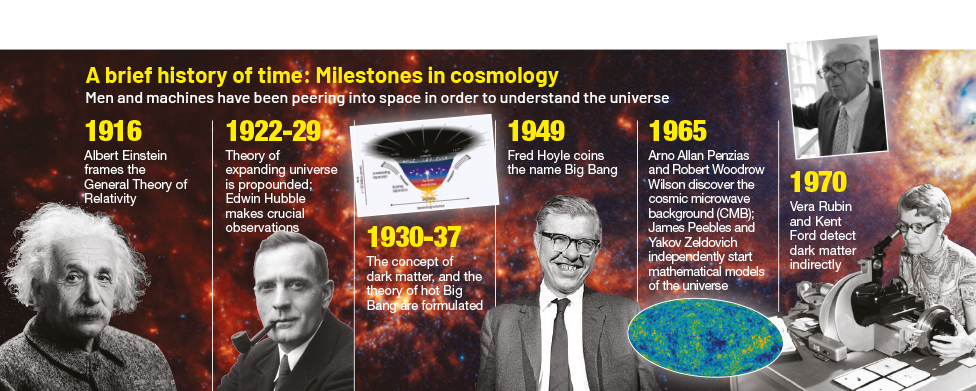
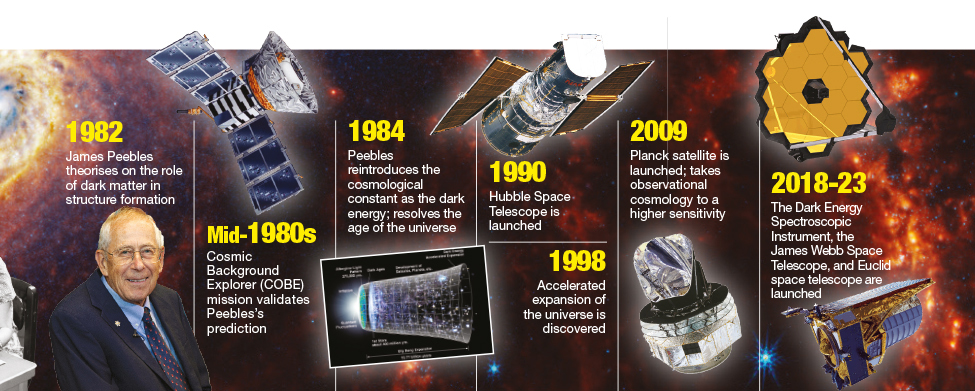
A NEW AGE OF ASTRONOMY
The Euclid mission had run into trouble almost immediately after launch. It was conceived by the ESA in 2007 as an ambitious programme to survey and catalogue objects visible over one-third of the night sky. This would mean measuring the shapes of two billion galaxies and the precise velocities of another 200 million. Since these objects are over large distances, and light from them takes a long time to reach the Earth, it is also like looking back over 10 billion years into the past. A large and precise catalogue will provide enough data for statistical analysis of the structure and evolution of the universe.
In September 2023, soon after the telescope reached the second Lagrangian point 1.5 million km from the Earth, scientists noticed ice forming on the instruments. This was from the water that remained in the spacecraft when it left the Earth. Engineers heated the spacecraft and evaporated the ice, but it returned in March this year, forcing a second and successful decontamination attempt. Meanwhile, the spacecraft had to deal with unexpected cosmic ray impact, and then scientists found that the telescope could not orient itself in space as was hoped. The orientation problem was solved through a software update. The mission was launched during high solar activity and therefore had to deal with more X-rays than anticipated, but scientists who analysed the consequences concluded that the impact would be minimal.
Over the next five years, astronomers around the world will get to work with large and sophisticated datasets.
Euclid started sending pictures in June this year, a few weeks before this edition went to print. It will continue to do so over the next six years, providing astronomers with a treasure trove of data. In March 2025, Euclid will release the first set of data, recorded for a small part of the sky, which is suitable for many types of astrophysics research but not cosmology. The next release will be in June 2026, and will mark the first big release of data suitable for cosmology. Two more releases are planned over its lifetime, one in 2028 and the other at the end of the mission. “The most fantastic thing about Euclid is that hundreds of scientists and engineers are working on the project,” says Roland Vavrek, Deputy Project Scientist of the mission, “with different backgrounds and from different countries. This is an incredible achievement.”
Astronomy missions, although developed by specific agencies, are often open to scientists all over the world. It means that over the next five years, astronomers around the world will get to work with datasets that are unprecedently large and sophisticated. It means that new ideas in cosmology, currently being discussed in the community, can be tested rigorously using real data. “A student starting out now will be part of a richer, more diverse and more data-driven cosmology community,” says Mohamed Rameez, a collaborator of Sarkar and a faculty member at TIFR.
See also:
Have a
story idea?
Tell us.
Do you have a recent research paper or an idea for a science/technology-themed article that you'd like to tell us about?
GET IN TOUCH